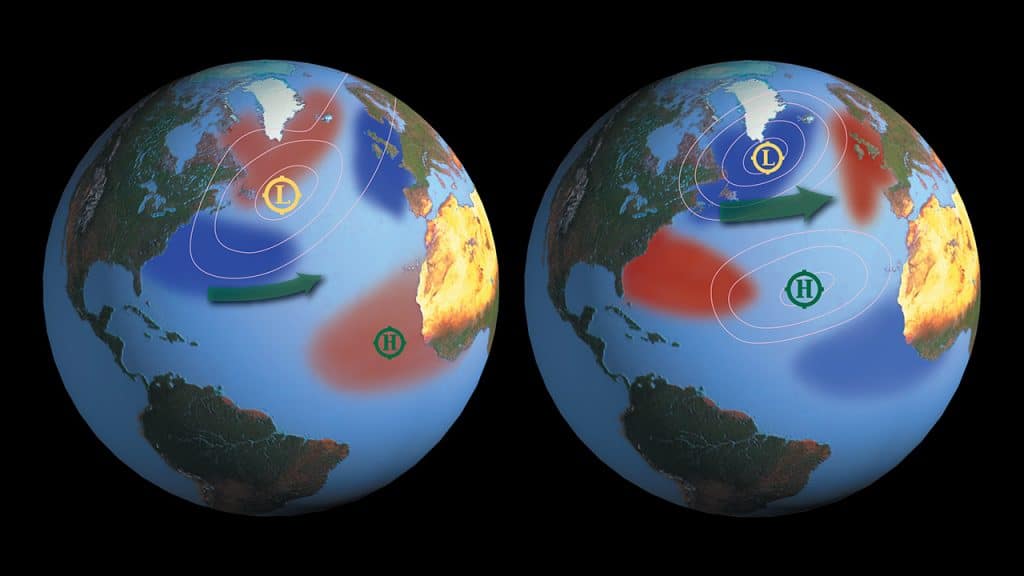
These global maps centered on the Atlantic Ocean show patterns of sea surface temperature during El Niño (left) and La Niña (right) episodes. The colors along the equator show areas that are warmer or cooler than the long-term average.
El Niño is a warming of surface waters in the eastern tropical Pacific Ocean. Together with, La Niña, these make up two of the three states of the constantly changing El Niño/Southern Oscillation (ENSO) that can affect weather patterns around the globe.
ENSO is just one of many oscillations in Earth’s ocean and atmosphere that occur naturally over different time and geographic scales. El Niño, Spanish for "the little boy" or "the Christ child," was named by Peruvian fishermen when they noticed changes in anchovy populations around Christmas more than 100 years ago caused by uncharacteristically warm surface water in the eastern tropical Pacific Ocean. Much later, scientists realized that El Niño was part of a much larger, recurring phenomenon that can bring about abnormal and often severe changes in temperature and precipitation throughout the tropics.
In a "normal," or ENSO-neutral year, a low atmospheric pressure center forms over northern Australia and Indonesia and a high pressure center forms on the other side of the Pacific over Peru. At the same time, the trade winds blow steadily east to west along both sides of the equator to move warm surface waters from the eastern to the western Pacific and cause cold, nutrient-rich bottom water to well up off the coast of South America.
La Niña, Spanish for "the little girl." As the name suggests, conditions this phase of ENSO are generally the reverse of El Niño. Where eastern tropical Pacific waters are warmer than normal during El Niño, they are much colder during a La Niña phase.
In an El Niño year, the high-pressure center over the western Pacific weakens, which diminishes or reverses the trade winds and permits the relatively weak east-flowing equatorial counter current to transport warm surface water towards South America. This reduces the cold upwelling along the coast, which can also diminish high-pressure over Peru and further weaken the trade winds.
During a La Niña event, the trade winds increase in strength, causing them to blow more warm water to the west and increasing the area of the eastern Pacific over which cold water wells up from deep beneath the surface. These conditions result in roughly opposite global weather patterns, some of which can be severe. In places where weather is exceptionally cool and wet during El Niño, it is generally hot and dry during La Niña. La Niña conditions are also associated with an increase in the number and strength of tropical storms in the Atlantic Ocean, whereas El Niño brings more severe tropical storms to the Pacific.
Once it has begun, an El Niño or La Niña event usually lasts about 12 to 18 months. The return to ENSO-neutral conditions begins in the southeastern tropical Pacific Ocean and gradually spreads westward. A La Niña event occasionally (but not always) follows an El Niño, and vice versa.
The Southern Oscillation is what is known as a "coupled" system because there is no clearly dominant driving force. Atmospheric conditions can (and do) influence the ocean and the ocean influences the atmosphere. Sometimes these influences amplify changes in one or more parts of the system (an effect known as a positive feedback), and other times they contradict or dampen changes (negative feedback). The result is a complex combination of interlinked changes that makes it difficult to predict the formation of El Niño or La Niña conditions with great detail.
The cycling of ENSO between El Niño and La Niña is irregular, but recurs about every two to ten years. This cycle is not a regular oscillation like the change of seasons; it is much more erratic in strength, timing, and pattern. Some decades have seen an ENSO event occur every few years while some decades have passed without even one. To identify El Niño (warm) and La Niña (cool) events within the Southern Oscillation, the National Oceanic and Atmospheric Administration now classifies ENSO events by the Oceanic Niño Index, or ONI.
Because of the wide variability in events leading to their onset, ENSO episodes are difficult to predict. For decades, scientists lacked the observational data that could be used in predictive models. However, widespread damage from the 1982-1983 El Niño event prompted a major study of the phenomenon. This 10-year program, named Tropical Ocean–Global Atmosphere (TOGA), developed an extensive oceanographic observation system to support climate studies. Since the completion of TOGA in 1995, the combined Tropical Atmosphere and Ocean (TAO) Triangle Trans Ocean Buoy Network (TRITON) project has continued to monitor the equatorial Pacific Ocean with a series of moored buoys. With this monitoring network in place, ENSO events are now often predictable up to a year in advance.
Many other naturally occurring ocean-atmosphere oscillations in the Pacific, Atlantic, and Indian Oceans have been recognized and named. Some of them have much more of an impact on climate and weather patterns in the U.S. and elsewhere than ENSO. Many of these, as during ENSO, ocean and atmosphere interact as a coupled system, with ocean conditions influencing the atmosphere and atmospheric conditions influencing the ocean. However, not all exert as strong an influence on global weather patterns, and some are even less regular than ENSO.
Antarctic Oscillation (AAO)
The Antarctic Oscillation (AAO) is a ring of variability that encircles the South Pole and extends as far north as New Zealand. It is characterized by a seesaw pattern in atmospheric pressure between the Antarctic region and middle latitudes between 40ºS and 50ºS. This results in alternating changes in wind and storm activity between these middle latitudes and higher latitudes near the southern oceans and Antarctic sea ice zone. In its positive (warm) phase, the AAO brings relatively light winds and more settled weather to middle latitudes, together with enhanced westerly winds over the southern oceans. In its negative (cool) phase, the westerlies are stronger over middle latitudes, with more unsettled weather, while windiness and storm activity ease over the southern oceans. The AAO is also referred to as the Southern Annular Mode (SAM).
Arctic Oscillation (AO)
The Arctic Oscillation (AO) is the Northern Hemisphere analog to the AAO and involves a similar seesaw pattern in atmospheric pressure between the North Pole and middle northern latitudes. Its negative (cool) phase brings higher-than-normal air pressure over the Arctic region and lower-than-normal pressure over the central Atlantic Ocean. These pressure differences leads to weaker westerly winds north and south of the equator. North of the equator, the weak westerlies allow cold Arctic air to reach farther south. During the cool phase, much of the U.S., as well as Northern Europe and Asia, experience cold and stormy winters. More storms develop over the Mediterranean region. The AO’s positive (warm) phase brings about the opposite conditions, with much of the U.S. and Northern Europe experiencing mild winter weather, and drought conditions prevailing in the Mediterranean. The AO and the North Atlantic Oscillation (see below) are collectively referred to as the Northern Annular Mode (NAM).
Atlantic Multidecadal Oscillation (AMO)
The Atlantic Multidecadal Oscillation (AMO) includes a series of long-duration changes in the sea-surface temperature in the North Atlantic Ocean. Like the Pacific Decadal Oscillation (see below), it has warm and cool phases that may last for 20 to 40 years at a time, with a difference of about 1°F between extremes. These seemingly small changes can affect the air temperature and rainfall over most of the Northern Hemisphere, particularly in the Atlantic region between the equator and Greenland, although some areas of the North Pacific may also be affected. Evidence suggests that Earth has been in a warm phase of the AMO since the mid-1990s.
Indian Ocean Dipole (IOD)
The Indian Ocean has its own seesaw behavior, the Indian Ocean Dipole. During a so-called positive phase, warmer-than-usual water temperatures in the western Indian Ocean bring heavy rains to East Africa and India and colder-than-usual waters bring drought to Southeast Asia. In the negative phase, ocean and monsoonal conditions reverse. (Illustration by E. Paul Oberlander, Woods Hole Oceanographic Institution)
The Indian Ocean Dipole (IOD) is characterized by an irregular oscillation of sea-surface temperatures in the eastern and western Indian Ocean. A positive or “warm” phase brings about greater-than-average sea-surface temperatures and greater precipitation in the western Indian Ocean region, along with a corresponding cooling of waters in the eastern Indian Ocean that tends to bring reduce precipitation in Indonesia and Australia. The negative or “cool” phase of the IOD brings about the opposite conditions, with warmer water and greater precipitation in the eastern Indian Ocean, and cooler and drier conditions in the west. The IOD also affects the strength of monsoons over the Indian subcontinent, with a negative phase corresponding to an increase in monsoon rains.
Madden-Julian Oscillation (MJO)
Named after Roland Madden and Paul Julian, who first described it, the Madden-Julian Oscillation (MJO) is a tropical disturbance that spreads eastward around the globe with a cycle of about 30 to 60 days and is the main oscillation behind weather variations in the tropics and subtropics. The MJO is most evident in the Indian and western Pacific Oceans, where it includes variations in wind, sea-surface temperature, cloudiness, and rainfall that, in turn, affect the intensity and break periods of the Asian and Australian monsoons. It can also interact with the Southern Oscillation, contributing to the intensity of an El Niño or La Niña event.
North Atlantic Oscillation (NAO)
The Indian Ocean has its own seesaw behavior, the Indian Ocean Dipole. During a so-called positive phase, warmer-than-usual water temperatures in the western Indian Ocean bring heavy rains to East Africa and India and colder-than-usual waters bring drought to Southeast Asia. In the negative phase, ocean and monsoonal conditions reverse. (Illustration by E. Paul Oberlander, Woods Hole Oceanographic Institution)
Many climate scientists consider the North Atlantic Oscillation (NAO) to be a regional manifestation of the Arctic Oscillation because both refer to similar climate phenomena. The NAO is a periodic change in atmospheric pressure between Iceland and Portugal that affects the strength of prevailing winds—the westerlies—over the North Atlantic Ocean, producing the strongest influence on weather patterns over the Northeast U.S. of any of the oscillations. These winds, in turn, affect the strength and direction of surface currents in the North Atlantic. During its "high-index" state, high atmospheric pressure develops over the Azores, and an intense low over Iceland. When the NAO index is high, ocean winds are stronger and winters milder in the eastern U.S. When the index is low, ocean winds are weaker and U.S. winters tend to be more severe.
North Pacific Gyre Oscillation (NPGO)
One of the most recent oscillations to be described, the North Pacific Gyre Oscillation (NPGO) affects the height of the sea surface in the Northeast Pacific. This, in turn, affects the intensity of the central and eastern branches of the North Pacific gyre—a major ocean circulation pattern in the North Pacific. Scientists have correlated the NPGO with previously unexplained fluctuations of salinity, nutrients and chlorophyll-a (algae) seen in long-term observations of the California Current and Gulf of Alaska. As a result, the NPGO may help scientists understand the mechanisms of primary production in the North Pacific Ocean that has a strong influence on the dynamics of the entire marine ecosystem in the region. Like the Pacific Decadal Oscillation (PDO), the NPGO fluctuates on the order of decades.
North Pacific Oscillation (NPO)
The North Pacific Oscillation (NPO) is a fluctuation of atmospheric pressure and sea-surface temperatures in the North Pacific Ocean that affects, among other things, winter temperatures over most of North America. The NPO is similar to the NAO in having a low-index state and a high-index state. A low NPO index is associated with southerly airflow along the west coast of North America, which tends to bring warmer air into the region. A high NPO index brings a northerly airflow, with the corresponding movement into the region of colder, sub-polar air.
Pacific Decadal Oscillation (PDO)
The Pacific Decadal Oscillation (PDO) is a long-term fluctuation that occurs in the Pacific Ocean every 20 to 30 years that is characterized by variable sea-surface temperatures in the north-central Pacific and near the Gulf of Alaska. The PDO mainly affects weather patterns in the U.S. Pacific Northwest. During its positive, or “warm” phase, a warm wedge of surface water forms in the eastern equatorial Pacific Ocean and a cool wedge forms in the northwestern Pacific. Its negative “cool” phase is characterized by a cold wedge in the eastern equatorial Pacific and a horseshoe pattern of warmer water connecting the northern, western, and southern Pacific. Most scientists agree that we are presently in a negative phase of the PDO. The most recent “positive phase appears to have lasted from 1977 to 1999.
Pacific-North American (PNA) Pattern
The Pacific-North American (PNA) Pattern relates the atmospheric circulation pattern over the North Pacific Ocean with the one over the North American continent through the height of the sea surface in the northern Pacific. By affecting sea-surface heights, the PNA causes strong fluctuations in air pressure and temperature in the region and beyond. Like other oscillations, it has two modes, positive and negative. The positive mode is associated with changes in the strength and location of the East Asian jet stream, above-average temperatures over western Canada and the western U.S., and below-average temperatures and drought conditions across the south-central and southeastern U.S. During the negative mode of the PNA pattern, there is a westward retraction of the East Asian jet stream, and a strong split-flow configuration over the central North Pacific. The western U.S. may experience relatively cold and wet conditions, while the eastern U.S. remains warm and dry during these negative modes.
When ocean and atmospheric conditions in one part of the world change as a result of ENSO or any other oscillation, the effects are often felt around the world. The rearrangement of atmospheric pressure, which governs wind patterns, and sea-surface temperature, which affects both atmospheric pressure and precipitation patterns, can drastically rearrange regional weather patterns, occasionally with devastating results.
Because it affects ocean circulation and weather, an El Niño or La Niña event can potentially lead to economic hardships and disaster. The potential is made worse when these combine with another, often overlooked environmental problem. For example, overfishing combined with the cessation of upwelling during an El Niño event in 1972 led to the collapse of the Peruvian anchovy fishery.
Extreme climate events are often associated with positive and negative ENSO events. Severe storms and flooding have been known to ravage areas of South America and Africa, while intense droughts and fires have occurred in Australia and Indonesia during El Niño events.
Changing weather patterns
Perhaps the most immediate and noticeable change is in the weather. The displacement of so much warm water in the eastern Pacific Ocean affects the amount of evaporation. Increased evaporation supplies more water vapor to the atmosphere and, consequently, results in greater precipitation on the neighboring land. Hence, countries on the western side of South America experience wetter-than-normal conditions, including severe storms during an El Niño phase of ENSO. Japan and western North America also experience stormier and warmer weather than usual. ENSO-related storms and flooding can wreak havoc in areas where climate is normally dry.
ENSO also has a direct effect on the formation of tropical storms and hurricanes. These become more frequent and intense over the Pacific Ocean. Violent tropical storms and hurricanes can produce gigantic ocean waves, which can swamp coastal areas and capsize ocean craft. In the Atlantic, El Niño may cause a quieter hurricane season, whereas La Niña events generally create conditions more favorable to Atlantic hurricanes.
Other parts of the world, which normally receive adequate or abundant rainfall, may instead experience damaging droughts or heat waves during El Niño. Researchers have found the strongest connections between ENSO and intense drought in Australia, India, Indonesia, the Philippines, Brazil, parts of Africa, western Pacific islands, Central America, and various parts of the United States. Drought occurs in each of these regions at different times and strengths during an El Niño event. Droughts can lead to uncontrolled forest or bush fires, crop failures, soil erosion, and desertification. Australia, Indonesia, East Africa, and parts of Brazil are also subject to intense heat waves during El Niños.
Cessation of upwelling
As mentioned earlier, the reversal of surface currents and consequently warmer waters in the eastern Pacific Ocean cause the ENSO-neutral upwelling of cold, nutrient-rich water to decline or stop along the coast of South America, and was the first sign of the workings of this oscillation in the ocean. This can have a serious impact on normally abundant fish stocks that exist along the western Pacific coastline. This water supports an entire food chain—from tiny green algae to large marine mammals, as well as human economic activity. When upwelling stops, the coastal water becomes warm and relatively nutrient-poor, forcing large numbers of important fish such as anchovies that depend on the algae to look elsewhere for food.
Damage to coral reefs
Abnormally warm water is known to cause serious damage to coral reefs. Shallow water corals are animals that live in a mutually beneficial, or symbiotic, relationship with tiny green algae that provide their host with energy. In response to high temperatures, many corals expel their symbiotic algae and turn white—a process known as bleaching. Lacking their algal partners, large stretches of coral reefs may die as their surroundings warm.
Impacts and severity
Naturally, the severity of an ENSO event determines its overall impact on the environmental. During strong ENSO events, a number of areas tend to be hard hit. The ENSO event of 1982–1983 was among the worst on record. Severe flooding and landslides occurred in the southwestern U.S., while Europe experienced abnormally cold weather. Major droughts affected large portions of Australia, Indonesia, China, India, Africa, and Central America while Peru experienced extreme damage from flooding and landslides. Corals were decimated across the equatorial Pacific Ocean. Food chains collapsed across the eastern Pacific coast.
Role of humans
While scientists continue to unravel the interconnected effects of individual and multiple oscillations on global weather patterns, it is becoming increasingly apparent that human activity is also behind observed changes in Earth’s climate. One of the most pressing questions arising from this realization is whether—and how—human-induced global warming will affect the changes caused by El Niño, La Niña, or phase of other oscillations.
This is most crucial for parts of the globe that experience such phenomena as El Niño-induced flooding or drought—especially because many places most heavily affected by such extreme weather events are often poor or able to only marginally support local populations. Scientists have only just begun to develop the actionable forecasts of weather related to El Niño and La Niña events to help people and governments prepare for abnormal events well in advance.