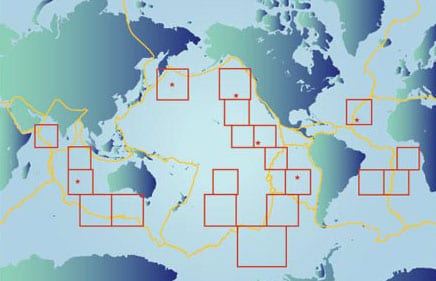
Ocean Seismic Network Seafloor Observatories
Our knowledge of the physical characteristics of Earth’s deep interior is based largely on observations of surface vibrations that occur after large earthquakes. Using the same techniques as CAT (Computer Aided Tomography) scans in medical imaging, seismologists can “image” the interior of our planet. But just as medical imaging requires sensors that surround the patient, seismic imaging requires sensors surrounding the earth.
Spring 1998 — Our knowledge of the physical characteristics of Earth’s deep interior is based largely on observations of surface vibrations that occur after large earthquakes. Using the same techniques as CAT (Computer Aided Tomography) scans in medical imaging, seismologists can “image” the interior of our planet. But just as medical imaging requires sensors that surround the patient, seismic imaging requires sensors surrounding the earth.
At the end of 1997 there were 105 high quality seismic stations on continents and islands around the globe as part of the US sponsored Global Seismic Network. In addition, smaller networks of land stations are sponsored by Japan and France. Ideally, seismologists would like to establish 128 seismic stations uniformly distributed over the surface of the globe. Why 128? Seismic processing, like processing on a personal computer, is more efficient if carried out with binary, that is, base 2, numbers. 128 is 2 to the seventh power. The next smallest power of 2, 64, would not give sufficient resolution of earth structure. The existing land-based networks are more dense than this. The next biggest power of 2, 256, would be simply too expensive. The 128 stations would be separated by about 2,000 kilometers. Since over two-thirds of the earth’s surface is covered by water, “uniformly distributed” implies that about 20 stations need to be located on the deep ocean floor, far from continents or islands. The goal of the Ocean Seismic Network (OSN) program is to develop the methodology and instrumentation necessary for continuous, high quality, seismic observations in the deep oceans.
There are three major challenges in establishing a seafloor seismic observatory: installing the sensor to obtain comparable quality to land or island stations, providing power to the instrument, and retrieving the data.
A ‘modern’ seismic installation will faithfully measure accelerations of the solid earth in three dimensions (vertical, north-south, and east-west) down to the quietest earth motions over a broad band of frequencies. In the absence of earthquakes and such human activity as traffic, shipping, and explosion tests, the earth’s surface vibrates in response to wind, ocean currents, and ocean waves. While the human ear responds to vibrations in air spanning frequencies from 20 hertz to 20 kilohertz, seismometers used to study whole earth structure respond to lower frequencies, from 0.002 hertz to 10 hertz. At 0.002 hertz the seismometer will rise and fall once in about 8 minutes.
Useful signals for tomographic studies of the earth have frequencies around 1 hertz. To get a feeling for the sensitivity of a modern seismometer, consider an earthquake and a receiving station that are 20° apart (about 2,200 kilometers or the distance from Woods Hole to New Orleans). A very large earthquake, with a magnitude of about 8, will cause displacements at the seismometer of about 1 centimeter. A small earthquake, with a magnitude of about 4, will cause displacements of about 10 millimicrons. (A millimicron is a billionth of a meter.)
The seismometer will also be responding faithfully to true earth ambient noise with frequencies around 1 hertz and displacements less than 1 millimicron. These correspond to accelerations of about one-tenth of a billionth of the acceleration due to gravity. Directly above a large, shallow earthquake, the surface of the earth will be displaced over a meter, but a high quality seismometer can measure displacements as small as a few millimicrons. The ability to faithfully measure small variations occurring at the same time as large variations is called dynamic range. A modern digital seismometer uses 24 bits to represent a number and has a “nominal” dynamic range of 144 decibels. If the full dynamic range were available, this would correspond to displacements ranging from a millimicron to about a centimeter. It is rare for a seismic station to be directly over a large earthquake. Special “strong motion” sensors are used for the very large displacements expected in earthquake-prone areas such as Japan and California.
A seismometer in Montana can measure ground vibrations that are excited by storms over the North Pacific and North Atlantic Oceans. Wave interaction during the storm at sea creates low frequency “sound” that travels through the solid earth to stations deep within the continents.
Experience on land with Global Seismic Network stations indicates that the best results are obtained by placing the seismic sensor in a borehole at about 100 meters depth. Although expensive, this has two advantages. First, a sensor set firmly in bedrock moves faithfully with the true motion of the solid earth. (Layers of soils, gravels, sands, and muds that lie on the hard rock basement distort the true earth motion.) Second, the ambient seismic noise levels, which effectively limit our ability to observe small earthquakes, are reduced considerably by getting away from the surface. The ambient noise, which is predominantly generated from atmospheric and oceanographic effects at the surface, is trapped in interface waves that attenuate rapidly with distance away from the interface. By getting below the region of interface waves, the ambient noise is considerably reduced. The International Ocean Network and Ocean Seismic Network communities are actively advocating the drilling of boreholes on the seafloor for seismic installations, and they are modifying the high quality borehole seismometers used on land for operations in the deep sea.
The digital data acquired by the borehole seismometer can be recorded on the hard drive of a seafloor computer. Data is acquired at 20 samples per second on each of three axes. At this data rate, a seismometer would fill the 1 gigabyte drive on a personal computer in about two months. Three approaches are being considered for data retrieval. Where retired submarine telephone cables cross an area, seismometers could be tapped into a seafloor junction box. Data could be retrieved and power could be supplied over the cable continuously in real time, an ideal situation. For remote areas without cables, the “brute force” approach is to record data autonomously in the seafloor package and grapple for the package periodically, say, once per year from a supply vessel. Batteries could be changed out at the same time. Though technically the simplest approach, the major disadvantage of this system is that the data would not be available until many months after the events of interest. The third idea is to moor, in the deep ocean near the seismometer, a buoy with a transmitter that can communicate via satellite to shore. This increases the power requirements considerably, but data could be available in near real time. However, maintaining a mooring continuously in the deep ocean is an expensive and challenging task.
A state-of-the-art broadband borehole sensor for deep sea applications draws between 4 and 12 watts of power continuously. (Compare this to leaving a 100-watt light bulb on in your home.) Improving the power consumption of deep sea seismometers and recording systems is an active engineering development task. A pressure housing 4 feet long and 12 inches in diameter filled with lithium batteries can run a 4-watt system for four months for about $10,000. Batteries alone for a 12-watt system would cost about $100,000 per year. Not inexpensive, but at least it can be done.
The Ocean Seismic Network Pilot Experiment
The primary goal of the Ocean Seismic Network (OSN) Pilot Experiment is to learn how to make high quality broadband (0.003–5 hertz) seismic measurements on the seafloor in preparation for extending the Global Seismic Network to the ocean basins. This experiment was carried out at a drill site (Ocean Drilling Program Hole 843B) 225 kilometers southwest of Oahu. In January and February 1998, we deployed three broadband seismic instruments: a borehole seismometer, a sensor buried surficially in the sediments, and a sensor resting on the seafloor. The seismometer was placed in the borehole using a Wireline Reentry System (see figure opposite). The instruments were recovered in June 1998.
While at the site in February, we acquired some sample time series data from each of the broadband seafloor installations. While tethered to the borehole sensor we recorded surface waves from the magnitude 6.1 event that occurred in Afghanistan on February 4 (top figure above). (The seafloor and buried sensors were not yet in place during this event.) Ambient noise spectra of the three components of the borehole sensor show that the horizontal components are noisier than the vertical components below about 0.04 hertz and that the horizontal components are quieter than the vertical components above the microseism peak at about 0.1 hertz (lower figure at left). Comparison of ambient noise in the borehole on the seafloor with a state-of-the-art sensor on the island of Oahu shows that seafloor stations have comparable noise levels to island stations (figure below) from about 0.02 hertz to 1.5 hertz. Scientists use these ambient noise curves and observations of signals from different sizes of earthquakes to evaluate the performance of a station.
Future Plans
The international community plans to install six OSN stations in the next five years. The Ocean Drilling Program has just completed a borehole on the Ninety-East Ridge in the Indian Ocean that will be used by the French for a borehole seismic station. At least one borehole station is planned near Japan. In fall 1998 a WHOI-led team installed a junction box on the retired AT&T cable between Hawaii and California, and ODP plans to drill a hole for the borehole seismometer at the site. There are also plans for two boreholes in the equatorial Pacific. The sixth site is on the Mid-Atlantic Ridge where a borehole already exists.
The goal of uniform seismic coverage of planet Earth is within sight, but we have a long way to go.
This work was supported by the National Science Foundation with additional support from Incorporated Research Institutions for Seismology, Joint Oceanographic Institutions, Inc., Scripps Institution of Oceanography, and a Mellon Independent Study Award from Woods Hole Oceanographic Institution.
Acknowledgments: The Ocean Seismic Network Pilot Experiment was a collaborative effort involving about 20 scientists, engineers, and technicians from Woods Hole Oceanographic Institution (WHOI) and Scripps Institution of Oceanography (SIO). In addition to the author, the principle investigators from WHOI were John Collins in the Department of Geology and Geophysics and Ken Peal in the Department of Applied Ocean Physics and Engineering. At Scripps the principal investigators were Fred Spiess and John Hildebrand from the Marine Physical Laboratory and John Orcutt and Frank Vernon from the Institute of Geophysics and Planetary Physics. Of course, the operations at sea also involved the officers and crews of the University-National Oceanographic Laboratory System research vessels. The deployment cruise was carried out on R/V Thomas G. Thompson (University of Washington) under the command of Captain Glen Gomes. Recovery was accomplished with R/V Melville (Scripps Institution of Oceanography) under the command of Captain Eric Buck.
Ralph Stephen’s Ph.D. thesis at the University of Cambridge was based on the first borehole seismic experiment carried out in the deep ocean in 1977, and he has been pursuing marine borehole seismology at WHOI ever since. In addition to this field work, Ralph develops synthetic seismogram methods for predicting sound propagation in the seafloor. His hobbies include being a husband and father.
Slideshow
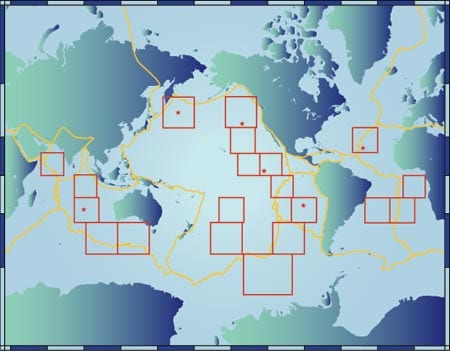
Slideshow
- This map shows 20 regions for locating seafloor seismic observatories in order to have 128 stations evenly spaced around the globe (red boxes). The six starred boxes have been selected as preliminary test sites. Yellow lines mark plate boundaries.
- The borehole seismometer deployed at OSN-1 sits on its "teeter-totter" on the fantail of R/V Thompson (University of Washington) in Honolulu Harbor.
- The autonomous seafloor data recording package for the borehole seismometer is deployed from R/V Thompson at OSN-1. The system is designed to acquire three channels of seismic data at 20 samples per second for four months.
- These seismic traces show the vertical vibration of the earth at Oahu (blue), OSN-1 (red), and southern California (green) caused by a magnitude 6.1 earthquake in Afghanistan on February 4, 1998. The earthquake event is the large amplitude arrival occurring in the middle of the traces. The left half of each trace shows the ambient noise prior to the event. In this figure the response at the OSN-1 observatory is better than at Oahu and comparable to Southern California.
- This "ambient noise spectrum" shows the magnitude of the earthA?s vibration in the frequency band from 0.001 hertz to 10 hertz for the vertical (Z) and two horizontal (H1 and H2) components of the borehole seismometer at OSN-1. The black dashed lines show the range of typical levels for land stations. Around 0.1 hertz and below 0.01 hertz for the horizontals, the seafloor station is as noisy as the noisiest land stations. Between 0.01 and 0.1 hertz on the vertical component and all components near 10 hertz the seafloor station is as quiet as the quietest land stations
- Procedure for installing the borehole seismic system in an existing borehole from a conventional, nondrilling, research vessel. The ship is maintained within a 10-meter watch circle by dynamic positioning and the Global Positioning System navigation. Acoustic transponders on the seafloor assist in locating the lead-in package relative to the re-entry cone. A camera and lights on the lead-in package at the bottom of the borehole seismometer also assist in locating the cone. Once re-entry is completed the system is lowered until the seafloor data recording package lands in the cone. The control vehicle has propulsion and additional navigational aids to manipulate the string near the seafloor. Once the system has been tested in place, the tether at the top of the recording package is released and the control vehicle returns to the surface. Recovery of the recording package is carried out with a grappling hook attached to the bottom of the control vehicle. At OSN-1 the water depth is 4,407 meters, the re-entry cone is 5 meters across, and the borehole seismometer was emplaced 248 meters below the seafloor.
Related Articles
- Can seismic data mules protect us from the next big one?
- Lessons from the 2011 Japan Quake
- Lessons from the Haiti Earthquake
- Seismometer Deployed Atop Underwater Volcano
- Worlds Apart, But United by the Oceans
- Oceanographic Telecommuting
- Rapid Response
- In the Tsunami’s Wake, New Knowledge About Earthquakes
- Ears in the Ocean