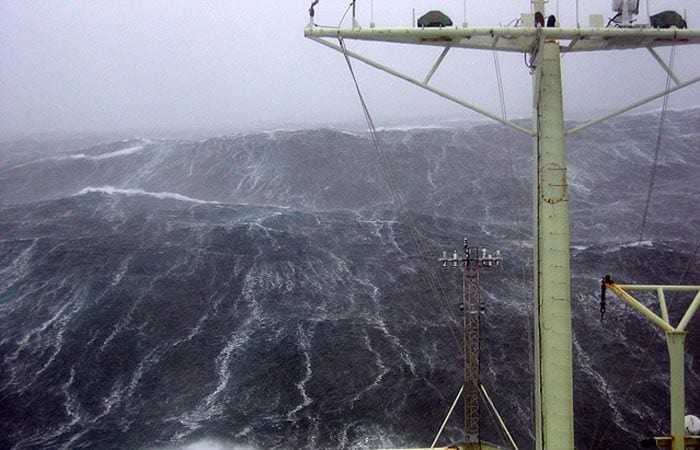
Into the Wild Irminger Sea
WHOI ship, scientists, and crew probe strategic oceanic gateway
In the Denmark Strait, Oct. 7, 2008
Maybe it’s lubberly to talk about those waves in the language of aesthetics, as if they were natural attractions like alpine peaks, but objective nautical numbers didn’t suffice. Were they running 10 meters? Higher. Fifteen?
The 55-knot wind gusted into the high-60s, once hitting 72, but the research vessel Knorr met the waves confidently, bow climbing skyward on the crests, easing down into the troughs. Endless three-story walls of blue-black water, white snakes of foam fleeing down their faces, breakers plunging from their crests.
This was a seascape of exquisite violence, heartlessly beautiful, a privilege to witness—from the safety of Knorr’s bridge. Capt. Kent Sheasley, Second Mate Derek Bergeron, the expedition’s chief scientist Bob Pickart, and a few others still ambulatory discussed the waves in hushed, respectful tones. We watched monsters and thought them wonders of nature.
The Denmark Strait separates Iceland from the east coast of Greenland by 250 miles of tough water. South of the strait, stretching from Iceland down to the latitude of Cape Farewell at Greenland’s southern tip, is the Irminger Sea—the windiest stretch of salt water on the globe. Though neither comes up very often in dinner-party conversation, the Denmark Strait and Irminger Sea comprise one of the most climatically significant and vulnerable regions in the world ocean.
Pickart, a physical oceanographer at Woods Hole Oceanographic Institution (WHOI), and other ocean scientists have been probing the Irminger for the past decade. Yet much about these mean waters remains unknown. No one aboard Knorr was surprised that we were hove-to studying nothing but the aesthetics of waves. But not only storms bedevil scientists and seamen in these seas. Their powerful, dizzyingly complex currents, convoluted seafloor topography, and tendency to freeze also challenge those seeking to comprehend these northern watery realms.
Kyle Covert, bosun of the WHOI-operated Knorr, came on the bridge with a damage report. A boarding sea had stove in a steel container mounted on the starboard side. Another boarding wave carried away one of the 2,000-pound mooring balls strapped to a stout rack welded to the deck. The straps didn’t tear; the wave ripped out the welds.
“Also,” said Covert, “the cover on that ventilator on the O-2 deck blew away. We’re taking water.”
We could see the ventilator in question—and the frozen spray on the O-2 deck—from the warm bridge. “Want me to go replace it now?” He was already suited up for battle.
“No,” said Capt. Sheasley, “I don’t want you to go out there.”
But of course he was going.
A chokepoint on the intersea highway system
How does water pass through the Denmark Strait? Where does it come from and how does it exit? These were the primary questions Pickart meant to address on this cruise. Neither is merely a pure-science matter of curiosity relevant only to subscribers of academic journals like Deep-Sea Research. What happens in the Denmark Strait and Irminger Sea matters to us all.
This narrow passage on the doorstep of the Arctic Circle is a bottleneck on what Pickart refers to as a “superhighway” in the oceans’ global circulation. It is the main route for waters flowing southward from the Arctic Ocean to the North Atlantic Ocean—a component of what is often referred to as the Ocean Conveyor.
Every second of every day, the North Atlantic Current, an offshoot of the Gulf Stream, transports about 15 million cubic meters of warm, highly saline water past the western shores of Britain and Ireland, cosseting those latitudinally incongruous palm trees in Cornwall. The North Atlantic Current “becomes” the Norwegian Current as it flows along that country’s coast, where it surrenders heat to the atmosphere, and in collaboration with the prevailing westerly winds, guarantees ice-free harbors in winter four degrees above the Arctic Circle. Together, these currents and their tributaries comprise the main highways bringing water northward through the Atlantic into the Arctic in the upper limb of the Ocean Conveyor.
If a certain quantity of water flows north, then an equal quantity must flow south. To keep the circle unbroken and the Conveyor turning, the ocean performs a unique trick. When the formerly Gulf Stream waters give up their heat, they become colder, and therefore denser, and sink to the depths in the deep basins of the Norwegian, Greenland, and Iceland Seas north of the Denmark Strait.
An oceanic gateway
Beneath the water in the Denmark Strait, shallow continental shelves, only several hundred meters deep, extend east from Greenland and west from Iceland and nearly join. They create a seafloor barrier separating the seas to the north and the Irminger Sea to the south, all of which are more than 2,000 meters deep.
Cold, dense water fills the depths of the seas north of the Denmark Strait and then plunges down an increasingly steep bottom slope into the depths of the Irminger Sea. Though not as abrupt as Niagara, this submarine waterfall is thousands of times greater in volume than all terrestrial waterfalls combined. If visible, it would be a World Heritage site.
As the cold, dense water sinks into the depths of the Irminger Sea, the ocean performs another trick: It pulls in surrounding water and marshals it into a vast current that flows southward at depth. The current eventually crosses under the Gulf Stream near Cape Hatteras, then flows seaward of the Bahamas, into the Southern Hemisphere. This is the Deep Western Boundary Current (postulated in 1960 by the eminent WHOI oceanographer Henry Stommel before it had ever been observed in nature).
Thus the Denmark Strait/Irminger Sea is at once the southbound superhighway of the Ocean Conveyor and the headwaters of the Deep Western Boundary Current. And that’s why we need to know how water navigates the strait.
A newly discovered ocean current?
In 2004, Icelandic oceanographers Steingrimur Jonsson and Hedinn Valdimarsson overturned previous thinking about how water enters the strait when they observed an entirely new current. They found that the old model was partly correct—the East Greenland Current flows into the strait from the north. However, they argued that most of the water that flowed over the Denmark Strait arrived via a deep, dense current flowing eastward along the northern border of Iceland. They called this deep current the Northwest Icelandic Jet.
Naturally, the new paradigm introduced more questions than it answered. Just what kind of water was contained in the Northwest Icelandic Jet? Where did it come from? How did it negotiate the submarine mountain ranges in its path?
To understand the net flow in the Northwest Icelandic Jet, Pickart needed to stop the ship every two miles to lower and retrieve a package of instruments called a “CTD,” which measures seawater conductivity (a proxy for salinity) and temperature at various depths. Then the ship moved to the next “station” to do it again. And again.
“The CTD operators probably want to throw me over the side,” Pickart would say later in the trip. “But there’s no other way.”
The second question—how water exits the strait—also brought a new twist to conventional thinking. Recent evidence suggested that some of the dense water passing out of the strait actually remains up on the outer portion of the shallow Greenland shelf, thus bypassing the big waterfall in the deeper regions of the strait.
Addressing this question required Pickart to raise his gaze from the deep ocean to the surface and above, to the atmosphere. The notion was that the wind might be driving water off the shelf into the deeper Irminger Sea basin. And in this case, terrestrial geography, in the form of Greenland, was enhancing the wind. This expedition provided us firsthand experience of the exquisite interconnectedness of nature’s great systems.
In the Denmark Strait, Oct. 10
The storm system moved eastward, as all eventually do; another weaker one followed, and another, like pearls on a necklace. We came to think of 40 knots as calm. One morning on the bridge, the captain, peering critically over a field of whitecaps, said, “You know what I hate?”
“What, Cap?”
“When shoreside weathermen say, ‘The storm passed safely out to sea.’ ”
Research-cruise routine slipped into its 24-hour-a-day, watch-on/watch-off pattern. Raw data poured in. Pickart and his graduate student, Kjetil Våge, sat 14 hours a day at their computers processing data from the CTD and another instrument called an Acoustic Doppler Current Profiler, or ADCP. This sonar-based device mounted in Knorr’s hull constantly pinged the water to yield measurements of current velocity.
Watch passed seamlessly to watch on the bridge and in the engine room. When the decks were dry, the bosun and his crew chipped rust, painted, and welded. The galley team turned out three square meals a day, two entrées for lunch and dinner, a total of 1,400 meals by the time the trip was through.
It depended on your watch schedule whether you saw in these waters and this weather only relentless gloom or something richer in Arctic character. The cloud formations are different here. So are the colors of sunrise and sunset, splintered as they strain through the murk. The very look of the ocean is different. But romantic day-workers, free from sleep deprivation and quite willing to fall under the spell of the Arctic, didn’t talk much about such things around the graveyard-watch standers.
Tools of the trade
To determine just how much water originating from the north flowed onto the shallow shelf in the Denmark Strait, Pickart extended some of his CTD lines all the way across the passage to within one mile of the Greenland coast. But to document how the dense water subsequently vacates the shelf farther south required more than a pass-over by a ship.
To record the shelf-to-basin transfer, Pickart and members of the WHOI Mooring Group had, during a cruise the year before, set seven closely spaced moorings astride the shelf break. The first order of business on this cruise had been to retrieve them and their year’s worth of data. As we neared their position in hopeful weather, Pickart explained the risk: Worst case, all were gone.
The moorings were armed with a WHOI-developed instrument called a Moored Profiler, a stout plastic pod housing temperature and salinity sensors and a current meter. The new wrinkle is that the Profiler chugs up and down the vertical mooring wire by means of a tiny traction motor, thereby collecting hundreds of measurements throughout the water column and throughout the year. If the ocean gives them back.
The strong local currents are quite capable of blowing down the moorings, even carrying them away. They can be disrupted by bottom trawlers that fish the area, and then there are icebergs the size of shopping malls marching over the mooring site. On the plus side, Pickart said, he had the best mooring team in the business working on the technical problems.
Led by WHOI senior engineering assistant Jim Ryder, the team snatched back all seven moorings in two Arctic-short days while the wind blew hard and snow accumulated on the pitching aft deck. Meanwhile, the bridge officers maneuvered the ship brilliantly. All of the profilers had survived the ocean’s onslaught.
But what about the data? Outwardly calm after a year’s wait, Pickart sat down at his computer and inserted a data-recording disc. The following morning, the verdict was in. The “ridiculously strong currents,” as Pickart put it, had broken four profilers, but not all at once. Each had lived long enough to capture precious data. Not a stellar survival rate, but quite impressive, given the woeful beating they’d incurred from the very forces they were laid to measure.
“It could have been far worse,” he said, relieved.
Kangerdlugssuaq Fjord, 68° N , Oct. 13
The East Greenland coast strains credulity. Utterly pristine, it seems more the work of some Arctic-besotted Romantic scene painter than the natural result of orogeny and glaciation. Equally unlikely, the sky was blue, blue, and the sea was dead flat.
As Oct. 13 dawned buttery-yellow, the snowy mountains glowed as if with some internal illumination. By noon, the light had turned starkly white, visibility unlimited. Pointed peaks, hollow cirques, and pyramidal mountainsides skirted by skree marched inland after soaring straight out of the deep blue water. A turquoise-veined iceberg, like a toppled high-rise, clogged the mouth of one nameless fjord.
Giddy as kids nearing our holiday destination, crew and science staff lined the port rail of the highest deck trying to capture it with cameras or simply take it in. No one lives here; no one ever has, and no one ever comes to this remote coast so hostile to human ambition. There is no flat land except on the glacier faces, no vegetation, nothing but rock and ice and ocean, Earth in its younger days.
We chugged northward at reduced speed, dodging growlers and bergy bits, to Kangerdlugssuaq Fjord on the eastern Greenland coast. The team laid a picket fence of CTD measurements across its mouth in blazing sunlight, while the rest of us watched Greenland’s snowy mountains and bergs go by.
After dinner, Pickart remarked that the CTDs were sampling water that could be traced back to remote places, including the Gulf Stream.
The Greenland Shelf, 69° N, Oct. 20
The bridge watch and visitors spotted a strange reflection just above the horizon. The radar had already seen it: sea ice athwart our desired course, and we were coming up on it fast.
Capt. Sheasley peeled off speed as we approached. Ice as far as you could see. He calculated his alternatives. It would take untold hours to detour around it, but it was new ice, still thin and slushy. The captain decided to drive through it—gingerly.
Silently, we watched and listened to ice scrape Knorr’s hull. And then we were past it. But then the ship sagged to a stop.
“Uh-oh,” said Capt. Sheasley before we understood what had happened. “The phone’s going to ring. It’ll be the engine room. I’m going to apologize profusely.”
The phone rang. The Cap apologized profusely for not informing the engine room that we were entering ice before it clogged the raw-water intakes. No harm done, we were soon under way. At dinner that evening, engineers offered snow cones straight from the engine intakes. No one ate any.
A savage storm
“URGENT: Please pay attention to strong storm low with winds of hurricane force. We recommend you to stay out of area between 19°W and 26°W and if possible seek shelter,” said the fax from the Denmark Meteorological Institute. We were at 20°W, where 80-knot winds, gusting to 100, with 40-foot seas, were expected—for the next 50 hours.
Those among us obsessed with the ocean wanted to stay to see the extreme show, and Capt. Sheasley admitted that it hurt his professional pride a bit to leave, but the only sensible decision, given the storm’s power and duration, was to make a run for it. In consultation with Icelandic colleagues ashore, he chose Eyjafjordur Fjord, a long crack in the north coast of Iceland 100 miles away. After three more uneasy CTD casts, we hauled in, battened down, turned on our heel, and bolted.
Dawn on Oct. 24 found us hunkered behind Hrisey Island with its tiny Christmas-card town near the mouth of the fjord. The weather map arrived. It was both frightening and thrilling. The isobars, those concentric rings of decreasing barometric pressure, were so tightly packed on the Greenland side they looked like a fingerprint.
The barometer would plummet 35 millibars in the next 24 hours, to a bestial 946 mb. Now we could see it: a towering, bulbous mass of night-black cloud plowing, like an island that had slipped its geologic moorings, over the mouth of the fjord. The wind sprang from two knots to 34, then to 55 knots—and we were in the lee of the island.
“Imagine what it must be like out there,” said the ocean obsessives.
Where wind, water, and land conjoin
Spinning counterclockwise around their centers of lowest pressure, autumn/winter cyclones track northeastward across the North Atlantic from Newfoundland, pass south of Cape Farewell at the southern tip of Greenland, and charge into the Irminger Sea region. Up in the northwest corner of our storm, hundreds of miles in diameter, hurricane-force winds were encountering the high, vertical coast of Greenland—an insurmountable barrier. The mountain wall bends the wind sharply southward, and the “squeezing” effect causes the winds to accelerate, like rapids in a narrow river gorge. This is called a “barrier wind,” and you could see it on the meteorological map in the smudge of isobars along the Greenland coast, where 90 knots prevailed.
As the barrier wind blows over the ocean’s surface, a basic principle of physical oceanography called “Ekman transport” takes charge of the actual flow. Influenced by the force exerted by Earth’s rotation, the net transport of water bends 90 degrees to the right of the wind direction in the Northern Hemisphere (to the left in the southern). It’s a law of physics. Here, however, the water cannot indefinitely bend to the right—because Greenland is in the way. Yet it has to go somewhere; it can’t defy the law.
In this case, the water gets forced downward, then seaward, likely pushing any dense water at the shelf’s edge into the deep Irminger basin. This wind-forced mechanism “perhaps” (Pickart’s prudent word) solves the riddle of how dense water that lingers on Greenland’s shelf eventually sinks to join the southerly flow of the Ocean Conveyor.
The biting irony aboard Knorr was that this storm, even now inflicting its will on the Irminger Sea circulation, was too powerful to allow us to measure it—or anything else. Though he took it like a pro, Pickart had lost almost two days during the first storm, another day while we fled from the second one, and three more hiding from its hurricane-force winds.
Now, as the scheduled end of the trip loomed, he’d lose still another day steaming back to the study area for one final CTD line. Pickart never expected, nor even wanted, gentle weather, but this was egregious, October being only the beginning of the storm season.
Nonetheless, between the Oct. 3 morning when we passed the Reykjavik sea buoy outbound and saw it again 28 days, 14 hours, 36 minutes later, we had covered 2,786 miles, retrieved seven moorings, and completed 263 CTD casts. Ignoring seasickness, we sustained no injuries, the ship no breakdowns.
And though Pickart, at this early stage, would not claim to know exactly how water enters and exits the Denmark Strait, he and his staff have acquired critical data. On a subsequent short research cruise, Pickart and his Icelandic colleagues continued measurements farther upstream and saw compelling new evidence for the existence of the Northwest Icelandic Jet and its role in feeding the deep overflow through the Denmark Strait.
He will need to return to these cold, violent waters again to elucidate the circulation in this strategic strip of ocean. That will take years, he predicts; there are few eureka moments in contemporary oceanography. However, sooner or later, the investigation continuing, questions will be resolved, and we’ll have learned a bit more about how the ocean system works.
This research was supported by the National Science Foundation.
Slideshow
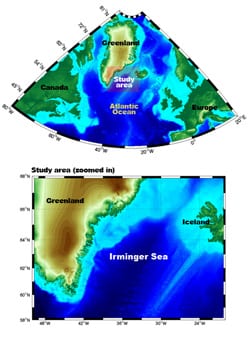
Slideshow
- The Irminger Sea is strategically located to play a critical role in the oceans' global circulation and the Earth's climate. But it has resisted extensive exploration, because it is one of the windiest and roughest patches of ocean on the planet.
- Scientists and crew aboard the research vessel Knorr faced winds ranging from 60 up to 100 knots and 30- to 40-foot tall waves on an expedition to the Irminger Sea in October 2007. (Photo by Kjetil V?ge, Woods Hole Oceanographic Institution)
- Waves wash over Knorr's decks in the dark of night during one of several fierce storms encountered during the research cruise. (Photo by Dan Torres, Woods Hole Oceanographic Institution)
- The North Atlantic Current carries warm, salty tropical surface waters northward and “becomes” the Norwegian Current. The currents surrender heat to the atmosphere, and prevailing winds help warm Europe in winter. As the waters give up their heat, they become colder, and therefore denser, and sink to the depths in the deep basins of the Norwegian, Greenland, and Iceland Seas north of the Denmark Strait. (Ruth Curry/Woods Hole Oceanographic Institution and Cecilie Mauritzen/Norwegian Meteorological Institute)
- The Greenland-Scotland Ridge looms like a great undersea barrier, stretching from East Greenland to Iceland and the Faroe Islands, and across to Scotland. The Denmark Strait is a critical checkpoint through which cold, fresher waters from northern seas flow across the ridge into the the main body of the North Atlantic Ocean. (Illustration by E. Paul Oberlander, Woods Hole Oceanographic Institution)
- Almost like a subsea waterfall, cold, dense waters flow southward over the Greenland-Scotland Ridge into the Irminger Sea and then underneath warmer, less dense waters. (Illustration by E. Paul Oberlander, Woods Hole Oceanographic Institution)
- Bob Pickart, a physical oceanographer at WHOI, was chief scientist of the Irminger Sea expedition. As well as exploring waters flowing through the Denmark Strait into the Irminger Sea, he also investigated the East Greenland Current flowing into the strait from the north and searched for evidence of a newly conjectured deep, dense current, called the Northwest Icelandic Jet, flowing eastward along the northern border of Iceland.
- To reveal the currents flowing and converging in the region, researchers stopped the ship every two miles to lower and retrieve a package of instruments called a “CTD," which measures seawater conductivity (a proxy for salinity) and temperature at various depths. Over the four-week cruise, crew and scientists did that 263 times. (Photo by Dan Torres, Woods Hole Oceanographic Institution)
- The researchers also used a WHOI-developed instrument called a Moored Profiler, a stout plastic pod housing temperature and salinity sensors and a current meter, deployed on a mooring that remains in the ocean for a year. The Profiler chugs up and down a mooring line by means of a tiny traction motor; it can collect hundreds of measurements throughout the water column and throughout the year. (Photo by Rick Krishfield, Woods Hole Oceanographic Institution)
- The Irminger Sea has another type of beauty when calm. Here the sun sets over an iceberg. (Photo by Nick M?ller)
Students from Seltjarnarnes Community in Iceland toured the WHOI-operated research vessel Knorr in early October 2008 before it departed for research in the Irminger Sea. The school was one of five from the U.S., Greenland, Iceland, and the United Kingdom that participated in an online expedition. During the voyage, students logged on to learn about oceanography and shipboard life by reading daily dispatches prepared in English and Greenlandic. Students also e-mailed questions to and received answers from scientists.
(Photo by Harpa Frimannsdottir)
Video
Related Articles
- Ocean in Motion
- A Hitchhiker’s Guide to the Ocean
- Detours on the Oceanic Highway
- A Newfound Cog in the Ocean Conveyor
- Mysteries at High Latitudes
- Floats Reveal Unknown Ocean Pathways
- Ocean Conveyor’s ‘Pump’ Switches Back On
- Submerged Autonomous Launch Platforms
- Will the Ocean Circulation Be Unbroken?
Featured Researchers
See Also
- The Irminger Sea: Where the Arctic meets the Atlantic A Web site with dispatches and information from the October 2007 research cruise.
- Robert Pickart's Homepage
- Research Vessel Knorr
- A Mooring Built to Survive the Irminger Sea from Oceanus magazine
- Adventure in the Labrador Sea An article by Bob Pickart on a Labrador Sea research cruise, from Oceanus magazine.
- Ocean Conveyor's 'Pump' Switches Back On from Oceanus magazine
- Reaching Up into Perilous, Icy Waters An article by Bob Pickart on the Arctic Winch in Oceanus magazine