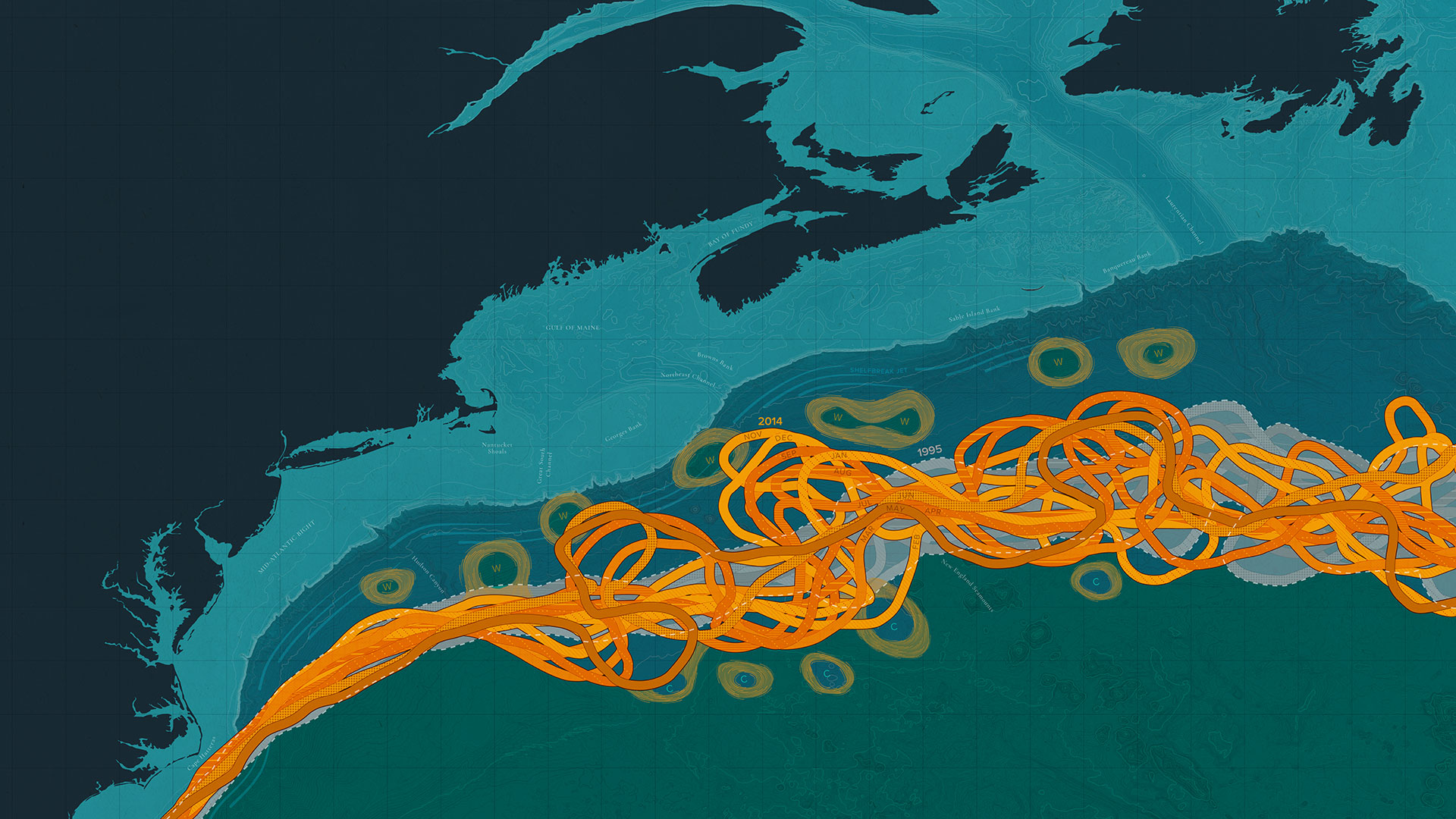
Ocean in Motion
How the ocean’s complex and chaotic physics define life on our planet
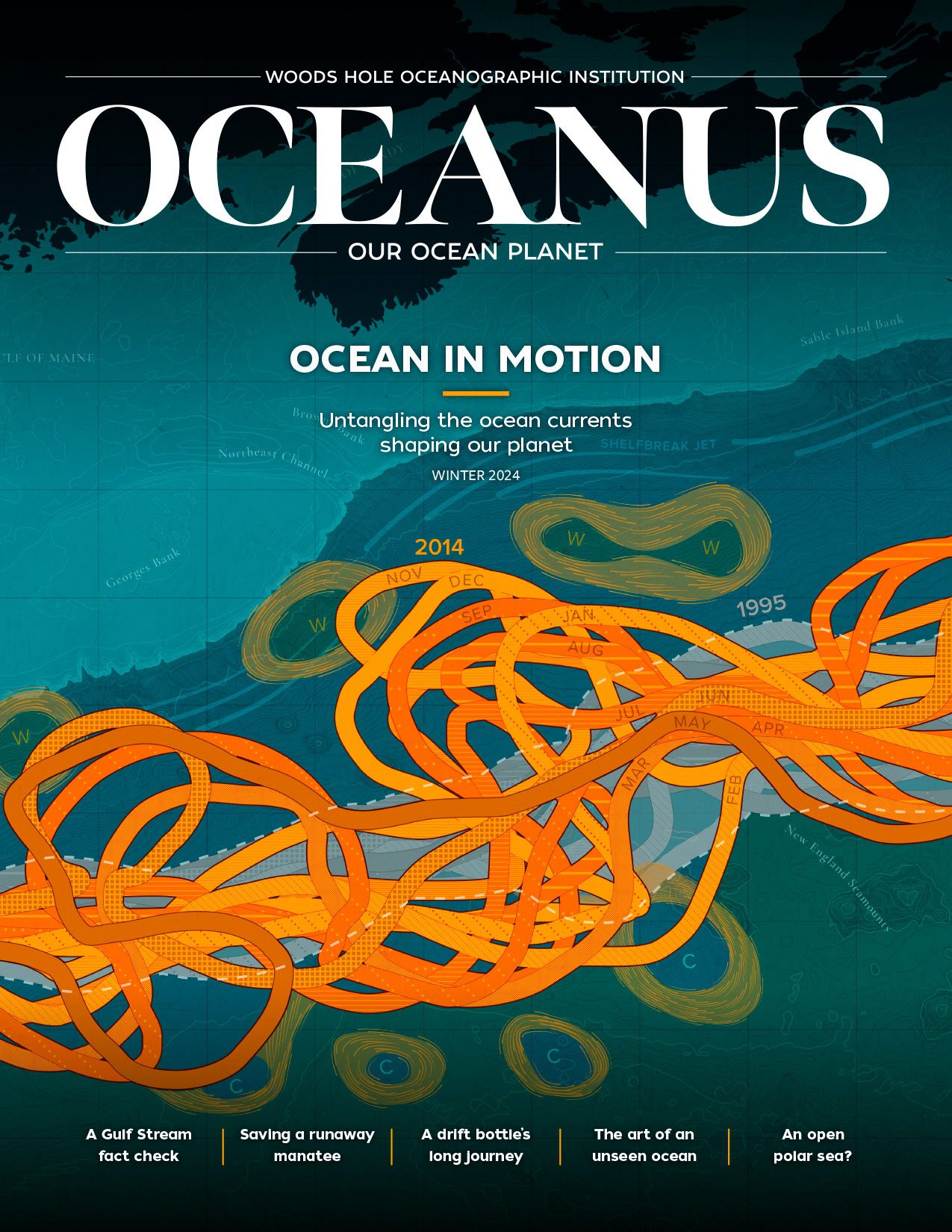
Estimated reading time: 14 minutes
Two thousand, five hundred pounds of syntactic foam hangs by a wire little thicker than a finger, and, with each roll of the ship, members of the research vessel (R/V) Endeavor fight to steady the massive mooring ball. From the observation deck, Nick Foukal, a physical oceanographer and adjunct scientist at WHOI, watches on, shoulders hunched against the chill of the Labrador Sea.
After a year’s delay, his 28-day expedition to map the flow and properties of the Labrador Coastal Current is off to an inauspicious start. Hurricane Idalia waylaid the ship in port and doubled the transit time to the mooring site. Now, in a thick fog, surrounded by the threat of icebergs, the crew will need to place seven moorings in three days before another forecast hurricane has the chance to overtake them. Miss this weather window and Foukal doubts they’ll have enough time to get all the instruments in the water. “I’ve been on ships before where we couldn’t complete all the objectives due to weather,” Foukal says. After years of planning, 10 days of rough travel, and hundreds of thousands of dollars already spent, there is a good chance the mission will come up short.
How to measure an ocean
Ask any physical oceanographer and they’ll tell you that measuring the ocean is no easy task. Each has a story of a storm or some unfortunate mishap that swamped their mission. Moorings crushed by icebergs. Instruments ripped up by wayward fishing trawlers. Critical tools and data lost to the deep. To the outsider, at least one with any sense of self-preservation or fear of seasickness, these arduous journeys can seem like a fool’s errand. But it’s critical work, says Bob Pickart, senior scientist at WHOI, who has spent his career braving rough seas and weather to map high-latitude oceanic processes. After all, he says, understanding the ocean’s complex and chaotic physics is key to understanding our place and future on the planet.
“Physical oceanography can be a pretty abstract science,” admits Pickart. “Sometimes it can be hard to wrap your head around.” From our terrestrial vantage point, we often forget that the ocean is a dominant force shaping the very nature of our planet. Much like our own circulation system, the ocean is a vast network of currents that shift staggering amounts of heat, nutrients, oxygen, CO2, and other chemicals throughout the entire globe. These properties are the foundational building blocks for everything from our global climate to regional weather to our food systems.
As the ocean churns, heat from the equator is distributed outward toward the poles; nutrients are upwelled toward the surface; oxygen is carried down to aerate the deep; even CO2 is extracted from the atmosphere and carted into the depths. The movement defines not just aquatic ecosystems but terrestrial ones as well.
“I’ve been on ships before where we couldn’t complete all the objectives due to weather.”
—Nick Foukal, physical oceanographer and adjunct scientist at WHOI
The magnitude of the ocean’s influence is bewilderingly large. In the North Atlantic, ocean currents shift 1 petawatt of heat from the equator northward to the pole. To put it into context, that is around 50 times the energy use of all humankind. Ocean currents are the reason London rarely has a white Christmas despite having a latitude on par with central Quebec’s. They’re the reason San Francisco can be a pleasant 65 degrees Fahrenheit (18 degrees Celsius) while Sacramento swelters in the hundreds. Ocean dynamics determine whether it will be a good year for wheat in India. They influence the intensity and location of life-giving monsoons in East Africa. They’re the reason fishing is good off the Grand Banks off Nova Scotia.
The ocean also traps 90% of atmospheric heat and absorbs almost 30% of CO₂ emissions from human activities every year. “It is really thanks to the ocean that the temperature of the atmosphere hasn’t increased to levels detrimental to human health,” says physical oceanographer Nathalie Zilberman, associate researcher at Scripps Institution of Oceanography and co-chair of the mission team for Deep Argo, an international program to measure the properties and physics of the deep ocean. “The ocean has very much been our biggest supporter in mitigating the effects of climate change.”
How water moves through the ocean tells us how heat is shifting around our planet, how CO₂ and oxygen cycle through the deep, how nutrients disperse throughout aquatic ecosystems. In turn, this information enables us to predict how sea levels will rise by the year 2100, how coastal ecosystems will look by mid-century, and what the average temperature will be like in a week, a year, or 50 years.
The ocean, however, is a non-linear and chaotic system, meaning nothing scales in a straight line, and even small perturbations in a current’s temperature, salinity, strength, or location can cause large changes that ripple through the planet’s teleconnection. Even in the face of this staggering complexity, physical oceanographers have made massive strides in understanding the ocean’s entangled physics. In the latter half of the 20th century, global profiling and mooring efforts helped chart large-scale ocean circulations, while in recent decades, expanded observation efforts have enabled scientists to uncover long-term trends in the Gulf Stream, reveal new complexities in the El Niño-Southern Oscillation, and peer even further into the deep ocean. In fact, says Zilberman, “in some regions of the deep ocean, we’ve collected more data in the past 7 years than the last 70 years combined,” referring to the ongoing achievements of the Deep Argo mission.
The advances have been remarkable, but even then, says Pickart, the ocean’s mysteries run deep. “There’s a heck of a lot we still don’t know about ocean processes and need to learn.” Parts of the ocean remain devilishly complex to measure, while some ocean dynamics operate on such lengthy timescales that it can take years or decades to accurately measure them. Other areas of the ocean are so remote and inaccessible that oceanographers are still just scratching the surface of understanding their dynamics. For instance, in 2008, Pickart and colleagues helped confirm the existence of a previously undiscovered current called the North Icelandic Jet, rewriting our understanding of the region’s flows.
In the global ocean, no current is too obscure; even small regional currents have been tied to drought, increased storm intensity, rising temperatures, and dwindling fisheries. Despite the Labrador Coastal Current’s relatively diminutive size, its changes have been linked to the slowdown of ocean-spanning circulation called the Atlantic Meridional Overturning Circulation (AMOC), which is considered a climate tipping point due to its ability to rapidly alter global climate patterns. The last time AMOC collapsed, around 12,000 years ago, temperatures across the North Atlantic dropped around 9 degrees Fahrenheit (~5 degrees Celsius) over the course of a few short decades. Yet, according to Foukal, “there’s very little knowledge about the Labrador Coastal Current at this point.” There have been individual moorings in the coastal current in the past, “but when you just have one mooring, it’s very hard to disentangle whether the current has strengthened or weakened, or if it’s moved laterally away from where your mooring is located.” But when the smallest changes can have massive impacts on global climate patterns, it’s critical that oceanographers continually refine and expand their observations to understand and predict changes.
Disentangling a complex chaos
Isabela Le Bras is no stranger to disentangling the ocean’s chaotic flow. As a seagoing physical oceanographer at WHOI, Le Bras focuses her work on how changes in the Arctic are affecting large-scale ocean dynamics.
“Everyone likes to think of the ocean like water in a coffee cup,” says Le Bras. “You move it around a little and everything mixes up.” But the ocean, she explains, is far from uniform. If you could visualize the different water masses in color, Le Bras says, you’d realize the ocean is more like slabs of water masses layered on top of one another colliding, sliding, twirling, wiggling, and flickering.
Each current and water mass has its own signature: a profile built from its temperature, salinity, density, and chemistry. Knowing these signatures helps determine where the water came from, how it will interact with other water masses, and how it may impact broader ecosystems as it moves across ocean basins. “Even as water flows over thousands of kilometers,” Le Bras says, “the properties can change surprisingly little.”
Measuring these signatures and tracking their path through the ocean is fine-tolerance science conducted at a massive scale, explains Le Bras. Oceanographers are not just trying to disentangle an invisible and complex web of currents at the surface, but also observe ocean processes hundreds or even thousands of meters deep. The ocean, however, is impenetrable to radar (radar’s electromagnetic waves are absorbed by water within a few feet of transmission), making it difficult for scientists to see beneath the surface. Instruments need to be placed on site, or in situ, deep beneath the waves in some of the planet’s most hostile and obscure corners, where subzero temperatures and crushing forces are the norm.
“The only way we can get a clear understanding of subsurface currents over a week or a month or a year is to keep our instruments in the water.”
—Susan Lozier, physical oceanographer at Georgia Tech
Ongoing visibility is critical to understand the daily, seasonal, yearly, and decadal variability of ocean dynamics, says Susan Lozier, physical oceanographer at Georgia Tech and the international project lead for OSNAP, an ocean-spanning observation system designed to measure ocean dynamics across the subpolar North Atlantic. “The only way we can get a clear understanding of subsurface currents over a week or a month or a year is to keep our instruments in the water,” she explains. Since 2014, the OSNAP array has used a series of moorings, gliders, and floats to provide a continuous record of the ocean’s flow, heat, and salinity in the region. But as the climate changes, so too are the very ocean dynamics that underpin our lives.
“We’re seeing patterns we haven’t seen before,” warns Glen Gawarkiewicz , WHOI senior scientist and physical oceanographer. Gawarkiewicz works with fishers and commercial entities in the Gulf of Maine to help understand how changing ocean dynamics impact their day-to-day operations.
When people think of changing ocean dynamics, they “tend to think on these really large-scale levels like AMOC and basin-scale changes,” says Gawarkiewicz. “But it’s really tricky when you get down to the scale of how ocean processes are affecting individual organisms and populations.”
Recently, in mid-May, fishers in the Gulf alerted Gawarkiewicz and colleagues to unusual fishing patterns in the region. When Gawarkiewicz and his team checked their array of instruments, they noticed that “the entire continental slope was three to five degrees centigrade cooler than usual.” This unusual cold pulse took him by surprise. In recent decades, the region has seen drastic changes in the frequency and magnitude of ocean processes. These changing dynamics “make it very hard to rely on past experience,” Gawarkiewicz says. The cold pulse not only impacted commercial fishing in the region but also changed the migration pattern of the critically endangered right whale, which in turn affected ongoing wind farm development in the region. As ocean dynamics change, we are beginning to see events that lie outside of our historical experience and expectations, says Gawarkiewicz. How these events will impact humans and ecosystems on a fine scale will be difficult to calculate.
A global ocean observation network
“If you’re going to observe the ocean globally, you’re not going to do it with the handful of ships that we have, especially with the existing the research fleet,” says WHOI physical oceanographer Steven Jayne.
Moorings and ships, Jayne explains, are only one part of a tapestry of tools scientists rely on to study ocean processes and measure the ocean’s changing properties. The ocean is too vast for ships and moorings to be operationally or financially feasible. In order to answer questions about the ocean’s circulation and permanently monitor its dynamics, sometimes you need to turn to other methods.
In an effort to expand both our temporal and spatial coverage of the ocean’s currents and properties, physical oceanographers are hard at work building a network of observation tools that can remain in the water 24/7/365 and operate at both the cost and the scale needed to cover the global ocean.
One of those programs is Argo, a global network of 4,000+ autonomous profiling floats which bob beneath the waves measuring ocean dynamics and properties. Thirty international partners contribute to the global array; as a member of Argo’s steering team, Jayne helps manage the U.S.-operated fleet. Every 10 days, a profile float returns to the surface, pinging its data back to oceanographers on land before slipping silently beneath the waves to continue its mission. As of 2020, Argo floats collect over 400 profiles a day from areas all across the globe, giving oceanographers on land a near-real-time look into subsurface ocean dynamics.
In the last two decades, the creation and rise of autonomous profiling floats and gliders have helped expand the traditional reach of shipboard surveys and mooring operations. Gliders and profiling floats are both cost-effective to use at scale and can stay in the water for months and years, says Jayne, especially in seasons and weather where humans have long since gone ashore. Weaving these new autonomous technologies together with mooring arrays, shipboard profiles, and satellite measurements, oceanographers have gained unprecedented insight into both surface and subsurface ocean dynamics. As profile and glider technology improves, scientists are utilizing it to expand their coverage of the ocean’s depths and remote regions. The result is an integrated network of observation tools that enables oceanographers to measure broad swaths of the ocean continuously and in near-real time.
“The ocean is a frontier and largely still an unexplored frontier.”
—Georgia Tech physical oceanographer Susan Lozier
In many ways, explains Jayne, oceanography’s growing observational network is mirroring the path that meteorology underwent in the mid-20th century, when scientists began developing large-scale systems for atmospheric observation. The networks enabled the real-time data collection necessary for modern-day weather forecasting and prediction.
Better coverage of ocean surface and subsurface dynamics means better model predictions, says Young-Oh Kwon, chair of WHOI’s Physical Oceanography Department. Alongside direct observation tools, models are a key part of our understanding of ocean physics. The two are closely entwined, says Kwon; “it’s a two-way street.” Direct observations help “ground truth the models,” meaning they help validate that a model mirrors real-world dynamics. Models, in comparison, help contextualize the observational data, fill in gaps in observational coverage, and extrapolate the data into both short-term and long-term forecasting.
“The goal,” says Jayne, “is to have a predictive system for the global ocean,” a true digital twin that synthesizes real-time data and advanced computational modeling to study, mirror, and predict the ocean’s complex and chaotic flows.
Still, there’s a long way to go before we completely understand all of the ocean’s complex and changing dynamics. The scale of the ocean’s influence is just so large. “When you compare the state of our knowledge to the importance, we are very behind in understanding the ocean,” says Kwon. But as observation networks continue to expand and our models become ever more refined, oceanographers are greatly improving our ability to predict changes throughout our planet: from long-term climate risks to the daily weather and ocean patterns that dictate our day-to-day lives.
A rising swell
Back on ship in the Labrador Sea, Foukal watches as one by one the moorings slide into the water. After the final mooring is deployed, the ship begins preparing for a rough night ahead. In the mess, Foukal hugs members of the mooring team and relaxes. There is still science to be done, more tests to salvage, but the primary objective, mooring the Labrador Coastal Current, is complete. In a year’s time he’ll retrace his steps, dodging hurricanes and icebergs, to recover the data.
Yet, as all physical oceanographers know, the ocean does not give up its secrets easily. “[To get] 100% data collection is rare,” Foukal says, “at least in the high latitude oceans.”
“The ocean is a frontier,” says Lozier, “and largely still an unexplored frontier.” It is vast. It is deep. It is full of corners, depths and dynamics yet to be fully explored. Yet in the patterns of its shifting and chaotic flows lie the answers to our place and future on the planet. Each new mooring, each new array, float, glider, model, and measurement unwinds the mysteries of the ocean a little further, until the future—our future—comes a little more clearly into view.
But sometimes, in the face of storms and icebergs, our pursuit of the ocean’s mysteries needs to wait.
Onboard the R/V Endeavor, Foukal watches as the swell begins to rise. Whatever questions he had about the ocean’s chaotic and dynamic flow are worries for another day. A storm is coming. The ship needs to run. After all, in this world, when it comes to our lives and the decisions we make, the ocean always has the final say.