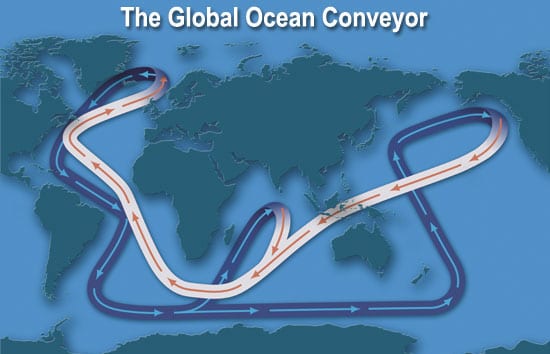
How the Isthmus of Panama Put Ice in the Arctic
Drifting continents open and close gateways between oceans and shift Earth's climate
The long lag time has always puzzled scientists: Why did Antarctica become covered by massive ice sheets 34 million years ago, while the Arctic Ocean acquired its ice cap only about 3 million year ago?
Since the end of the extremely warm, dinosaur-dominated Cretaceous Era 65 million years ago, heat-trapping greenhouse gases in the atmosphere have steadily declined (with the anomalous exception of the last century), and the planet as a whole has steadily cooled. So why didn’t both poles freeze at the same time?
The answer to the paradox lies in the complex interplay among the continents, oceans, and atmosphere. Like pieces of a puzzle, Earth’s moving tectonic plates have rearranged themselves on the surface of the globe—shifting the configurations of intervening oceans, altering ocean circulation, and causing changes in climate.
The development of ice sheets in the Southern Hemisphere around 34 million years ago seems fairly straightforward. The supercontinent of Gondwana broke apart, separating into subsections that became Africa, India, Australia, South America, and Antarctica. Passageways opened between these new continents, allowing oceans to flow between them.
When Antarctica was finally severed from the southern tip of South America to create the Drake Passage, Antarctica became completely surrounded by the Southern Ocean. The powerful Antarctic Circumpolar Current began to sweep all the way around the continent, effectively isolating Antarctica from most of the warmth from the global oceans and provoking large-scale cooling.
The Northern Hemisphere is more problematic. From sediment cores and other data, we know that until about 5 million years ago, North and South America were not connected. A huge gap—the Central American Seaway—allowed tropical water to flow between the Atlantic and Pacific Oceans.
A growing body of evidence suggests that the formation of the Isthmus of Panama partitioned the Atlantic and Pacific Oceans and fundamentally changed global ocean circulation. The closing of the Central American Seaway initially may have warmed Earth’s climate, but then set the stage for glaciation in the Northern Hemisphere at 2.7 million years ago.
The Ocean Conveyor
A fundamental element of today’s climate system is a conveyor-like ocean circulation pattern that distributes vast quantities of heat and moisture around our planet. This global circulation is propelled by the sinking of cold, salty—and therefore dense—ocean waters.
In today’s ocean, warm, salty surface water from the Caribbean, the Gulf of Mexico, and the equatorial Atlantic flows northward in the Gulf Stream. As the warm water reaches high North Atlantic latitudes, it gives up heat and moisture to the atmosphere, leaving cold, salty, dense water that sinks to the ocean floor. This water flows at depths, southward and beneath the Gulf Stream, to the Southern Ocean, then through the Indian and Pacific Oceans. Eventually, the water mixes with warmer water and returns to the Atlantic to complete the circulation.
The principal engine of this global circulation, often called the Ocean Conveyor, is the difference in salt content between the Atlantic and Pacific Oceans. Before the Isthmus of Panama existed, Pacific surface waters flowed into the Atlantic. Their waters mixed, roughly balancing the two oceans’ salinity.
About 5 million years ago, the North American, South American, and Caribbean Plates began to converge. The gradual shoaling of the Central American Seaway began to restrict the exchange of water between the Pacific and Atlantic, and their salinities diverged.
Evaporation in the tropical Atlantic and Caribbean left ocean waters there saltier and put fresh water vapor into the atmosphere. The Trade Winds carried the water vapor from east to west across the low-lying Isthmus of Panama and deposited fresh water in the Pacific through rainfall. As a result, the Pacific became relatively fresher, while salinity slowly and steadily increased in the Atlantic.
As a result of the Seaway closure, the Gulf Stream intensified. It transported more warm, salty water masses to high northern latitudes, where Arctic winds cooled them until they became dense enough to sink to the ocean floor. The Ocean Conveyor was rolling, drawing even more Gulf Stream waters northward.
Revving up the Gulf Stream
How does this make ice in the North?
Peter Weyl hypothesized in 1968 that the closure of the Central American Seaway and the intensification of the Gulf Stream would have brought a critical ingredient for ice sheet growth to the Northern Hemisphere—moisture. Weyl’s theory assumed that the closure of the Central American Seaway and the buildup of salt in the Atlantic coincided with the growth of northern ice sheets between 3.1 and 2.7 million years ago.
But doubts about this hypothesis surfaced in 1982, when Lloyd Keigwin found evidence in ocean sediments that the closing of the Isthmus of Panama had influenced ocean circulation more than a million years earlier. He demonstrated that the salinity contrast between the Atlantic and Pacific had already started to develop by 4.2 million years ago.
In 1998, Gerald Haug and Ralf Tiedemann confirmed Keigwin’s research with higher-resolution data from sediment cores. If the salinity had already changed by 4.2 million year ago, why didn’t glaciation start until 2.7 million years ago? On the contrary, the Earth experienced a warm spell between 4.5 million and 2.7 million years ago.
That global warm spell, called the Mid-Pliocene Warm Period, may also have been related to the closing of the Central American Seaway and the consequent rearrangement of global ocean circulation. An invigorated Ocean Conveyor could have driven a stronger flow of deep waters from the Atlantic to the North Pacific Ocean, which is the end of the line for deep-ocean circulation.
On their journey to the North Pacific, these deep waters became enriched in nutrients and carbon dioxide. In the Subarctic Pacific, these deep waters could have upwelled, rising to the sunlit surface to provide the ingredients to spark enormous blooms of phytoplankton. Great abundances of silica and opal (the preserved material from the phytoplankton shells) in seafloor sediments are evidence of both the blooms and the strong upwelling.
The upwelling may have been so strong, however, that the phytoplankton did not keep pace with the upwelling—that is, more carbon dioxide upwelled than was used by the phytoplankton. Consequently, the excess carbon dioxide “leaked” back into the atmosphere, adding a greenhouse gas that warmed the planet.
Short-circuiting the Conveyor
What shut down the Mid-Pliocene Warm Period about 2.7 million years ago? And what finally caused Northern Hemispheric glaciation at about the same time—but nearly 2 million years after the Isthmus of Panama formed?
Weyl’s original theory of a stronger, moisture-laden Gulf Stream raised another nettlesome question: How could the Gulf Stream—which transports not only moisture but also heat to the North Atlantic—lead to major Northern Hemisphere cooling and the formation of ice?
Neal Driscoll and Gerald Haug proposed one solution. They postulated that moisture carried northward by the Gulf Stream was transported by prevailing westerly winds to Eurasia. It fell as rain or snow, eventually depositing more fresh water into the Arctic Ocean—either directly, or via the great Siberian rivers that empty into the Arctic Ocean.
The added fresh water would have facilitated the formation of sea ice, which would reflect sunlight and heat back into space. It would also act as a barrier blocking heat stored in the ocean from escaping to the atmosphere above the Arctic. Both these phenomena would further cool the high latitudes. In addition, Arctic waters flowing back into the North Atlantic would have become less cold and salty—short-circuiting the efficiency of the Ocean Conveyor belt as a global heat pump to North Atlantic regions.
The tilt toward glaciation
These preconditions—moisture plus an Arctic nucleus for cooling—would have made the climate system highly susceptible to ice sheet growth. Even modest changes in the global environment would have been sufficient to tip the scales and lead to the onset of major Northern Hemisphere glaciation.
Just such a change occurred between 3.1 and 2.5 million years ago, as Earth’s axis fluctuated so that the planet’s tilt toward the sun was less than today’s angle of 23.45 degrees. Less tilt to the Earth would have reduced the amount and intensity of solar radiation hitting the Northern Hemisphere, leading to colder summers and less melting of winter snows.
The onset of Northern Hemisphere glaciation also affected the Subarctic Pacific. It led to the formation about 2.7 million years ago of a freshwater lid at the surface of the ocean, called a halocline. This Arctic halocline would have created a barrier to upwelling, which blocked deep carbon-dioxide-rich deep waters from rising to the surface. The “leak” of heat-trapping carbon dioxide into the atmosphere was stemmed, further cooling the planet.
Many other ocean-atmosphere feedback mechanisms, resulting from the opening and closing of oceanic gateways, remain imperfectly understood. And scientists are also exploring the ramifications of other oceanic gateways.
Mark Cane and Peter Molnar, for instance, have suggested that the uplifting and movement of the Indonesian Islands between 5 and 3 million years ago would have fundamentally re-directed less warm South Pacific water and more cooler North Pacific water through the Indonesian Seaway. The consequence might have been that the Pacific changed from more permanent El Niño-like conditions (which move heat from the tropics to high latitudes) to a more La Niña-like state (which would have curtailed the heat transfer and cooled the Northern Hemisphere).
The lessons from these vast geologic and geographic changes is both elegantly simple and excruciatingly complex. The opening and closing of seaways has a profound influence on the distribution of fresh water, nutrients, and energy in the global ocean. The coupling of these changing oceans with a changing atmosphere inevitably means a changing climate.
Slideshow
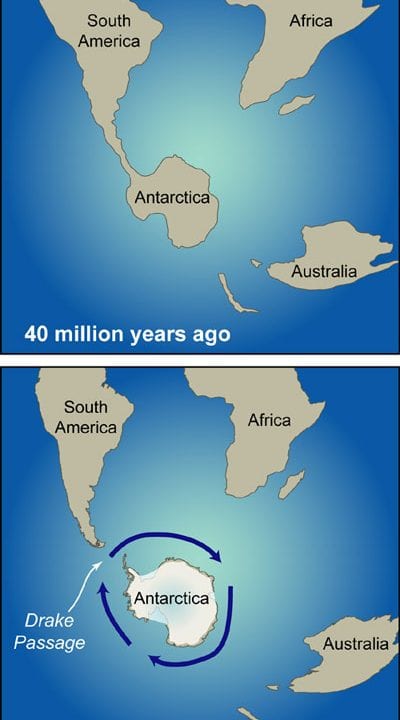
Slideshow
- How Antarctica got its ice sheets —In the continual movement of Earth’s tectonic plates, Antarctica was severed from the southern tip of South America about 34 million years ago, creating the Drake Passage. Antarctica became completely surrounded by ocean. The powerful Antarctic Circumpolar Current began to sweep around the continent, isolating Antarctica from the warmth of the global oceans and provoking large-scale cooling.
- Today’s climate system is influenced by the ocean’s conveyor-like global circulation. Cold, salty waters sink to drive the conveyor, and warm surface currents complete the loop. (Jayne Doucette.)
- Surface waters flowed from the Pacific into the Atlantic 10 million years ago via an ocean gateway called the Central American Seaway, and both oceans had the same salinity.
- About 5 million years ago, the North American, South American, and Caribbean Plates converged. The rise of the Isthmus of Panama restricted water exchange between the Atlantic and Pacific, and their salinities diverged. The isthmus diverted waters that once flowed through the Seaway. The Gulf Stream began to intensify.
- Today, evaporation in the tropical Atlantic and Caribbean leaves behind saltier ocean waters and puts fresh water vapor into the atmosphere. Trade Winds carry the water vapor westward across the low-lying isthmus, depositing fresh water into the Pacific through rainfall. As a result, the Atlantic is saltier than the Pacific. (Illustration by Jack Cook, WHOI)