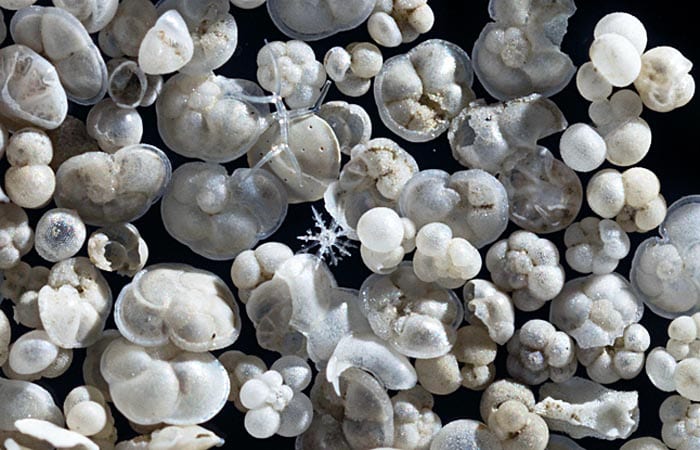
A Tale of Two Oceans, and the Monsoons
Tiny seafloor shells could reveal big clues to the forces that generate monsoons
Every summer, the continent of Asia takes a big breath. This inhalation pulls moisture-laden air from the Indian Ocean over India and Southeast Asia, causing torrential rains known as the monsoons. For as long as there have been people in India and Southeast Asia, lives have been set by the rhythm of the monsoons.
Some years, too much rain brings devastating floods and mudslides; in other years, too little rain causes droughts and famine. In 2007, flooding displaced about 30 million people from their homes. Over the past 200 years, several droughts have each caused more than 5 million deaths.
If we could forecast upcoming monsoon seasons, we could take steps to avoid death and destruction. But the monsoons have defied prediction so far, partly because they are generated by complex interactions among land, air, and two oceans. Predicting the monsoons will become even more difficult as global warming creates a climate that we have not experienced in recorded history. We won’t be able to use our current climate as a guide to the future.
But we can use the past. One way to unravel the complexities of the monsoon system is to study how it has worked in the past. To do this, we use some very small shells, preserved in sediments at the bottom of the sea, to answer some very big questions about climate.
A seesawing ocean
During the summer, the huge landmass of Asia heats up like a brick in the sun; hot air rises over the continent, and cool ocean air, filled with moisture, flows in to replace it. During the winter, the air over the Indian Ocean is warmer than the air over land; the ocean air rises, drawing cool dry air from Asia out to sea.
The size of the air mass that Asia breathes in depends, in part, on the temperatures of the Pacific and the Indian Oceans. And the temperatures of these oceans are influenced by two large-scale, dynamic patterns of winds and water temperatures that seesaw between two extremes: El Niño-Southern Oscillation (or ENSO) and the Indian Ocean Dipole.
During “normal” years in the Pacific Ocean, trade winds blow along the equator from Central America toward Indonesia, pushing warm surface water toward the western Pacific. In the eastern Pacific, cool water from the deep ocean is pulled up to the surface to replace the water blown to the west. The constant blowing of the trade winds maintains a Pacific Ocean whose surface is tilted. So much warm water piles up in the west, the sea surface height there is about 7 inches higher than in the east.
Occasionally, the trade winds will weaken just for a bit. Without the trade winds holding the mound of warm water in place, it begins to slide back to the east. This is an “El Niño” event. As the pool of warm water from the western Pacific spreads eastward across the Pacific, it creates a cap of warm water in the east, effectively preventing the cool deep water from reaching the surface.
How the monsoons work
During El Niño years, the entire Pacific is warmer than usual. And that can take Asia’s breath away during the summer. A hotter Pacific Ocean can effectively compete with the hot Asian landmass, so that no cool, moist air is drawn landward, and ultimately, the monsoons fail over India.
The sea surface temperature in the Indian Ocean is a mirror image of the Pacific; it is warm in the east and cool in the west. The Indian Ocean has its own seesaw behavior, the Indian Ocean Dipole. During a so-called positive Indian Ocean Dipole event, warm waters in the east spread toward the west, somewhat like in an El Niño. The winds that blow along the equator also switch directions and blow toward the west. These westward-blowing equatorial winds enhance monsoon winds and create a more powerful monsoon. India’s largest rainfall in the past 150 years occurred during a positive Indian Ocean Dipole event.
Predicting the monsoons is so difficult because ENSO and the Indian Ocean Dipole seem to take turns influencing the monsoons. In some years, an El Niño event has a strong influence on the monsoons, and the Indian Ocean Dipole has none. In other years, it is vice versa. The reasons for this trade-off are not entirely clear, but it probably has to do with the exact timing, strength, and positioning of the warm pools during El Niño and Indian Ocean Dipole events.
The past holds clues to the future
In our quest to study past climate, our main clues come from foraminifera. These are amoebas, but unlike the tiny blobs you saw in the pond water in junior high school, these amoebas build shells around themselves, made from calcium carbonate. The shells contain evidence of seawater conditions when the foraminifera were living and building their shells.
A foraminifer lives for about a month. When it dies, it sinks to the seafloor. It falls on top of the dead foraminifera from last year, and next year’s foraminifera will fall on it. Research vessels can collect a core of these sediments, with a large tube inserted into the seafloor. Back in the lab, we can extract and analyze foraminifera shells to reconstruct ocean conditions, and we can date the shells to determine when those conditions were occurring. Together, these let us create records of climate conditions that extend many thousands of years into the past.
Back on land, the cores are sliced open. We scope out slices of mud with a little spatula. When the cores are fresh, the consistency of the mud is similar to frosting. We rinse the mud in a sieve so that the sample is mostly shells. With the naked eye, the shells look like grains of sand. Under a microscope, they are beautiful, shaped in spirals, disks, spheres, tubes, and cones. There are shiny shells and pitted shells and even the occasional pink shell.
From the core mud, I pick out two species of foraminifera, G. ruber and P. obliquiloculata, and move them to a separate dish with a very fine paintbrush. G. ruber lives right at the surface of the ocean, while P. obliquiloculata lives deeper, at approximately 300 feet.
If both G. ruber and P. obliquiloculata record cooler temperatures, it indicates that cold water from the deep has been brought all the way to the surface. If just the deeper-dwelling P. obliquiloculata record cool temperatures, this could indicate that cold, deep water has not made it all the way to the surface. Perhaps it was blocked by a buoyant cap of fresh water, suggesting increasing monsoonal rainfall. While measuring two species is twice the work, knowing the temperature of both surface and subsurface waters is important if we want to understand how the mechanisms of the climate system interact.
Elementary evidence
We analyze the shells’ chemistry in a mass spectrometer, investigating ratios of carbon, oxygen, calcium, and other elements that substituted for calcium in the shell while it was growing. Variations in these elements can tell us about the temperature and salinity of the seawater that existed when the foraminifera were alive. They can also tell us about past levels of freshwater runoff into the ocean from rivers; increases indicate times in the past when monsoonal rainfall has risen.
With my Ph.D. advisor Delia Oppo, I am examining sediments that have been cored from the seafloor in the eastern Indian Ocean, along the coasts of the islands of Java and Sumatra. This location was picked because ocean conditions there are influenced by ENSO, the Indian Ocean Dipole, and the monsoons. By examining several cores from this region, we hope to tease out how these three systems interact.
Our goal is to create climate records for the past 25,000 years, a time span that encompasses many different climate conditions. It includes a time when much of North America was covered by an ice sheet and a time when the global climate was slightly warmer than it is today. That will give us the next-best way to understand how our climate system works under a variety of conditions, because we can run only one experiment at a time on Earth’s climate—the one we are running now.
Fern Gibbons has been supported by The J. Seward Johnson and The Arthur E. Maxwell Graduate Student Fellowships. Her research was funded by the National Science Foundation and the Ocean and Climate Change Institute at WHOI. This article was written during a science writing course for graduate students at WHOI, supported by funds from The Henry L. and Grace Doherty Professor of Oceanography.
Slideshow
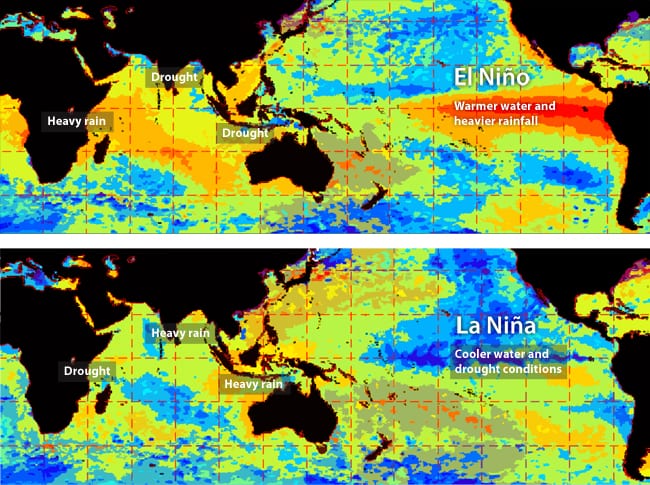
Slideshow
- The strength of the Asian monsoons has been linked to natural oscillations in the Pacific Ocean. When surface temperatures in the eastern equatorial Pacific are warmer than usual (an El Niño event), heavy rains hit East Africa and droughts beset India, Indonesia, and Australia. When ocean conditions flip-flop (a La Niña), so do rainfall patterns across Asia.
- The Indian Ocean has its own seesaw behavior, the Indian Ocean Dipole. During a so-called positive phase, warmer-than-usual water temperatures in the western Indian Ocean bring heavy rains to East Africa and India and colder-than-usual waters bring drought to Southeast Asia. In the negative phase, ocean and monsoonal conditions reverse. (Illustration by E. Paul Oberlander, Woods Hole Oceanographic Institution)
- MIT/WHOI graduate student Fern Gibbons extracts tiny preserved shells of marine life from sediments cored from the seafloor. The shells hold chemical clues that reveal ocean conditions in the past and suggest how they might change in the future. (Photo by Tom Kleindinst, Woods Hole Oceanographic Institution)
- The seafloor sediments are full of fossilized microscopic shells whose chemistry can reveal ocean temperatures that existed tens of thousands of years ago. (Photo by Tom Kleindinst, Woods Hole Oceanographic Institution)