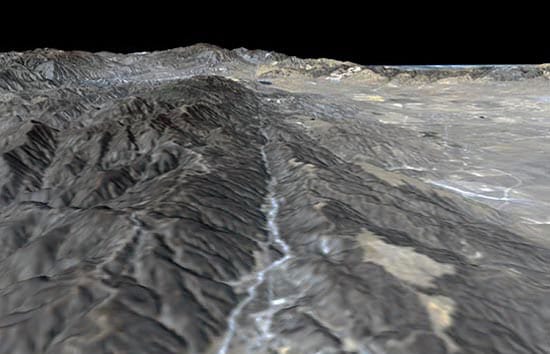
Earthshaking Events
New research on land and sea reinvigorates hopes of forecasting where earthquakes are likely to occur
When I was still a schoolboy in China, two major earthquakes occurred, about a year apart. They had a profound impact on my life and on the Chinese people.
The first was a quake with a magnitude of 7.3, which struck the northern city of Haicheng on Feb. 4, 1975. For six months before the quake, a series of much smaller quakes had rumbled in the region. They accelerated on Feb. 3, and in the early morning of Feb. 4, the government began to issue warnings, shutting down factories and urging people to remain outdoors. Despite the frosty weather, people moved into open fields, where children watched movies instead of going to school. The shock arrived at 7:36 p.m. While more than one million dwellings were badly damaged, the quake’s death toll was low at 1,328. The foreshocks had offered a warning signal, and this amazingly successful earthquake prediction probably saved tens of thousands of lives.
Seventeen months later, the people of Tangshan, a city 280 miles southwest of Haicheng, were not so lucky. On July 28, 1976, a magnitude 7.8 earthquake released the energy equivalent of 400 Hiroshima atomic bombs combined. There were no foreshocks, and therefore no warning.
The quake struck at the worst time, 3:42 a.m., when most people were sleeping in their beds. It lasted only about 90 seconds, but about 90 percent of the houses and buildings in Tangshan collapsed. A total of 242,769 people died, and 169,851 were severely injured, according to an official tally.
Bamboo and body bags
The Tangshan earthquake occurred during a political era when China shrouded itself from outside eyes. So the devastation it wrought did not make headlines in the West. But it left an imprint on me and other Chinese that is perhaps as profound and indelible as September 11th on the current generation.
My home province in southern China harbors bamboo forests, and much of our harvest was sent to Tangshan to construct emergency shelters. I also recalled vividly that factories in my home city made a huge quantity of large plastic bags to be sent to Tangshan. So many people died, those plastic bags were needed to bury the dead.
Undoubtedly, these events encouraged me to become a geophysicist and earthquake researcher—to seek to understand the fundamental physics of earthquakes and learn, for example, why foreshocks preceded the quake in Haicheng, but not in Tangshan. Underlying all our scientific efforts is the goal of forecasting earthquakes and a desire to save lives.
In many ways, earthquake research is like cancer research: They are scientific challenges that offer huge potential benefits to society, yet both have turned out to be far more complex and intractable than we thought and hoped they would be. But scientists have made important advances in cancer research, and I believe that new seismological research on land and in the oceans has us on the path to make similar advances in seismology.
Location, location, location
We know, in general, where earthquakes typically occur. They happen near faults, or fractures in the earth’s crust where rock formations—driven by the inexorable movements of Earth’s tectonic plates—grind slowly against each other and build up stress. At some point, stress surmounts friction, and the rocks slip suddenly, releasing earthshaking seismic energy.
We have a general idea where major faults are near Earth’s tectonic plate boundaries. Many faults in California are named, including the famous and highly visible San Andreas Fault, which stretches from north of San Francisco to northern Mexico. But there are many, much smaller, and less well-known faults that might prove even more dangerous because they are closer to, and even directly underneath, urban centers such as Los Angeles. In many places, including right beneath Los Angeles, faults don’t break the surface. We have only vague ideas about the locations of these so-called “blind faults,” until surprising earthquakes illuminate their existence.
Forecasting the timing of earthquakes is more difficult. Our best estimates are currently based on the general theory that faults accumulate strain and slip on somewhat regular schedule. This isn’t very useful for very large faults and great earthquakes, which require centuries of strain build up before they rupture.
From Joshua Tree to Hector Mine
In our quest to forecast earthquakes, scientists have searched for any signs of precursors that might provide warning. Even a few minutes’ warning would be useful, providing enough time to stop trains, shut down nuclear power plants, or turn off the gas in your house, for example.
Scientists have investigated changes in groundwater levels and electromagnetic fields and unusual animal behavior before major quakes, but the evidence so far, though intriguing, is often complex and not conclusive. Seismologists are also examining changes in seismic patterns that may foretell large quakes—an increase in smaller earthquakes, as happened before Haicheng, or perhaps a dying out of smaller earthquakes immediately before the Big One.
With colleagues Ross Stein of the U.S. Geological Survey (USGS), Geoffrey King of Institut Physique du Globe in France, and Andrew Freed of Purdue University, I have explored an intriguing seismic pattern in southern California that we believe can improve our capability to identify areas that are more susceptible to future earthquakes. Our research has been supported by the National Science Foundation (NSF), the USGS, and the Southern California Earthquake Center.
Since 1992, a sequence of four moderate to major earthquakes has occurred in the Mojave Desert—near Los Angeles, but fortunately in sparsely populated, outlying regions. In April 1992, a magnitude 6.1 quake struck near the town of Joshua Tree, on a small fault about 20 kilometers east of the San Andreas Fault. Two months later, on another fault about 30 kilometers north, a magnitude 7.3 quake occurred in Landers—followed just three hours later by a magnitude 6.3 quake near the town of Big Bear.
Do quakes “talk” to each other?
In the wake of these earthquakes, we calculated how rocks shifted and deformed. We constructed a three-dimensional computer model that simulated how stress was transferred throughout the rocks in the regions, from the brittle upper crust to the more fluid-like lower crust. The model pointed to an increase in pressure of 1 to 2 bars in the direction of the town of Hector Mine, about 20 kilometers northeast of Landers.
One bar is equivalent to the pressure exerted by pushing your hands together. That’s not much, but applied over a wide area that is on the verge of a quake, it adds up. In 1999, a magnitude 7.1 hit Hector Mine. We believe the Landers and Big Bear quakes transferred stress, through the crust and the slowly creeping upper mantle, toward the Hector Mine fault and hastened its eventual failure seven years later.
Our research indicates that as an earthquake alleviates stress on one fault, it can shift that stress to adjacent areas. In a domino-like effect, strain can “creep” through the crust, interact with neighboring faults, and trigger another earthquake elsewhere.
In this way, earthquakes carry on a conversation with each other. If we listen in, we can begin to understand and monitor how one quake can influence the timing and location of earthquakes on faults “down the line.”
Subsequent research on fault systems in Turkey and Japan have shown that these concepts of “creeping” and “stress triggering” may give us a valuable new tool to assess where earthquakes are more likely to happen next. We believe, for example, that the Landers and Hector Mine quake sequence together has shifted stress toward the San Bernardino Mountain segment of the San Andreas Fault and increased the likelihood of a quake there.
Faults under the oceans
Simple explanations for earthquakes on land have been difficult to unravel because continental crust is old and usually has a long, complex, and tortured history. It is a conglomeration of many types of rocks that have been distorted by erosion and chemical “weathering” over tens to hundreds of millions of years; overwritten by many periodic bursts of volcanism; and repeatedly fractured and refractured by earthquakes.
These confounding complications have encouraged seismologists to study a simpler system that naturally creates earthquakes—on the bottom of the ocean, where the majority of Earth’s tectonic plates collide and where about 80 percent of all seismic activity on Earth takes place. Many of the plate borders are marked by volcanic mountain chains called mid-ocean ridges, where new ocean crust is created. The mid-ocean ridges are offset, in a zigzag pattern, by great transform faults, which resemble faults on land.
The seafloor offers many advantages: ocean crust that is pristine and composed of only a few types of rocks; fault systems that are uncomplicated and undistorted; and far more seismic activity to observe. By studying seafloor systems, we have the potential to discover fundamental aspects about earthquakes that are applicable to land.
Eavesdropping on oceanic quakes
Studying earthquakes in the oceans presented its own difficulties in the past. Beyond the usual expenses and logistics of seagoing research, seismometers previously could not stay down long enough to capture seismic events and could not record a broad range of seismic waves. But the growing potential of long-term ocean observatories, new generations of seismic instruments, and exponentially increased computer power are dramatically increasing our chances of making seismological breakthroughs.
Previous experiments by researchers have showed tantalizing evidence of precursory “slow” quakes before a major quake on oceanic faults. Instruments installed on land recorded a very slow creeping event 100 seconds before a magnitude 7.0 quake in 1994 on the Romanche Transform Fault in the equatorial Atlantic Ocean, a fault very similar to San Andreas. A similar experiment in the Prince Edward Transform Fault in the Indian Ocean showed a slow creeping event 15 seconds before a magnitude 6.8 earthquake.
In 2004, funded by NSF and the WHOI Deep Ocean Exploration Institute, we analyzed seismic data collected by the National Oceanic and Atmospheric Administration (NOAA) since 1996 along the Siqueiros Transform Fault, an 80-mile fault sandwiched between two volcanic mid-ocean ridges in the Pacific Ocean. NOAA’s seismic instruments are suspended in the SOFAR (sound fixing and range) channel, an exceptionally good zone of sound transmission that is 700 to 1,500 meters beneath the sea surface. Earth shaking from even a small quake in the ocean crust can vibrate into the SOFAR channel and be recorded by NOAA instruments hundreds of kilometers away.
Twin quakes
The NOAA instruments recorded many small quakes on the Siqueiros Transform Fault. Trish Gregg, a graduate student in the MIT/WHOI Joint Program, Deborah Smith, a senior scientist at WHOI, and I read the records carefully, and we were surprised! Beginning in early May of 1998, several small earthquakes began, culminating in a magnitude 5.8 quake on May 10.
As we did in California, we calculated how this main shock changed the stress field in the region. In the areas where we calculated that the stress was transferred, smaller quakes continued to occur. Then, another flurry of smaller quakes began to occur in an outlying area about 20 kilometers away, followed by another magnitude 5.8 quake in that area only 18 hours after the first one.
Flurries of foreshocks also appear to have occurred prior to a number of other moderate-size earthquakes in our study region in the Pacific. These new findings give us confidence that we can figure out how foreshocks are created and that we can calculate how and where one earthquake can transfer stress and trigger another earthquake nearby. The research reinvigorates hopes that at least some earthquakes on Earth have relatively high predictability.
A rising phoenix
Almost three decades after its devastating earthquake, Tangshan is a flourishing new city. Its population has increased by more than 50 percent since 1976. Tangshan was one of the first Chinese cities honored by the United Nations for its hospitable residential environments. A new city landmark—the Phoenix Hotel—rises 112 meters into the sky and is specially designed for resisting shakes from major earthquakes.
However, the people of Tangshan are fully aware of the constant danger posed by a very complex network of faults in northern China. Recently, I began to collaborate with colleagues to evaluate how the Haicheng, Tangshan, and other moderate-size quakes in the region may have “talked” to each other. We hope to assess more accurately seismic risks for the Beijing-Tianjing-Tangshan triangle, the true cultural and political heartland of China—so that the future will bring us more Haichengs and fewer Tangshans.
Slideshow
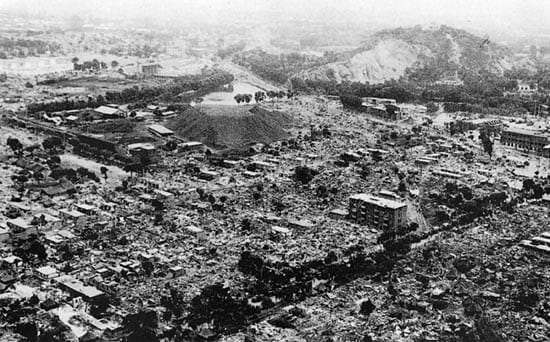
Slideshow
- Without warning, a magnitude 7.8 earthquake struck the city of Tangshan in China, killing 242,769 people and destroying 90 percent of the city's buildings. A similar earthquake struck 17 months earlier about 280 miles northeast near Haicheng. But a series of smaller foreshocks provoked Chinese officials to issue warnings urging people to remain outdoors, and only 1,328 people died. (Courtesy: China Stock Photo Agency)
- Two major earthquakes occurred 17 months apart in the mid-1970s near the cities of Haicheng and Tangshan in northern China. (Illustration by Jack Cook, WHOI Graphic Services)
- Faults on land, like the San Andreas Fault near Palmdale, California (top), are similar to those in the ocean, like the Atlantis Transform Fault in the Atlantic Ocean (bottom, three times vertical exaggeration). But in many ways, oceanic faults are easier to study and offer the potential to discover fundamental aspects about earthquakes that are applicable to land. (Top credit: NASA/JPL/NIMA; bottom credit: Tom Reed, University of Hawaii, and Brian Tucholke, WHOI)
- WHOI geophysicist Jian Lin stands near the plainly visible ruptured surface of the Pleasant Valley Fault in Nevada, which caused a magnitude 7.1 earthquake in 1954. (Photo courtesy of G. Valensis/Italian Institute of Seismology)
- A satellite image shows a portion of the San Andreas Fault and smaller neighboring faults (white lines) in southern California. (Courtesy: NASA/JPL/NIMA)
- Since 1992, a sequence of four moderate to major earthquakes has occurred in sparsely populated places in the Mojave Desert, near the towns of Joshua Tree, Landers, Big Bear, and Hector Mine. (Courtesy of Andrew Freed, Purdue University)
- Computer models simulated how the Joshua Tree, Landers, Big Bear, and Hector Mine quakes alleviated stress in some places (blue areas) and transferred and increased stress (red areas) to adjacent regions. In a domino-like effect, strain can "creep" through the crust, interact with neighboring faults, and trigger another earthquake elsewhere. Scientists believe that the Landers and Hector Mine quake sequence together have shifted stress toward the San Bernadino Mountain segment of the San Andreas Fault and increased the likelihood of a quake there. (Courtesy of Jian Lin, WHOI)
- In early May of 1998, hydrophones began to record a flurry of small earthquakes near the Siqueiros Transform Fault, an 80-mile fault sandwiched between two volcanic mid-ocean ridges in the Pacific Ocean. The flurry culminated in a magnitude 5.8 quake on May 10. This was followed by another flurry of smaller quakes in an outlying area about 20 kilometers away and another magnitude 5.8 quake only 18 hours after the first one. New findings like this reinvigorate hope that further understanding of foreshocks can provide warning of larger quakes, at least in some places on Earth.
- A computer model simulated how stress on rocks is transferred in a region. At left, rocks on either side of a fault push against each other, building up stress. At right, the stress overcomes friction, and the fault ruptures violently in an earthquake. In the aftermath, strain is alleviated in some areas (blue) and transferred and increased to other neighboring areas (red). (Courtesy of Ross Stein, USGS)
Related Articles
- Can seismic data mules protect us from the next big one?
- Lessons from the 2011 Japan Quake
- Lessons from the Haiti Earthquake
- Seismometer Deployed Atop Underwater Volcano
- Worlds Apart, But United by the Oceans
- Oceanographic Telecommuting
- Rapid Response
- In the Tsunami’s Wake, New Knowledge About Earthquakes
- Ears in the Ocean