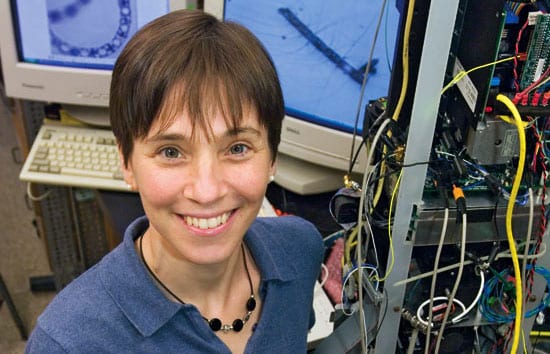
Building an Automated Underwater Microscope
A conversation with biologist Heidi Sosik
Heidi Sosik studies marine phytoplankton—the community of microscopic plants that essentially make all the food for all the ocean. Like an anthropologist documenting an undiscovered society, Sosik wants to learn everything she can about the living things in her community—their seasonal activities, nomadic habits, and nutrition. She is also part engineer, building and tinkering with gadgets that promise to bring the microscopic world of phytoplankton into focus.
Sosik is the first scientist to receive a joint fellowship from two WHOI Ocean Institutes, the Coastal Ocean Institute and the Ocean Life Institute. This summer and fall, she and colleagues are testing a new instrument, the Imaging FlowCytobot, at the WHOI Martha’s Vineyard Coastal Observatory (MVCO).
Very few people are aware that the majority of the world’s plants live in the ocean. Why are they so important?
Phytoplankton are like the grass and trees of the ocean. They’re just as important to the planet as land plants, but basically invisible—unless you go looking for them with tools and technologies such as the ones I use. It’s easy to forget that they’re there, but it would be a disaster for us if we forgot, and thus neglected to take care of the ocean—a huge portion of the planet.
The water over the continental shelf harbors an entire ecosystem. Phytoplankton are plant-like cells that feed larger plankton organisms and the offspring of larger animals. And this begins a web of interactions from plankton to the fish, whales, and birds that feed on them.
What we’re after is a better understanding of what factors in the environment determine where and when different kinds of phytoplankton grow and bloom.
What are some of those ecologically important factors?
Phytoplankton are photosynthetic, so they need sunlight to make organic matter. And they need nutrients, essentially fertilizer. All the compounds that you read on the side of your breakfast cereal box—iron, vitamins, and nitrogen—phytoplankton need those things, too.
Typically, nutrients are concentrated in the deep waters of the ocean. But a variety of ocean circulation patterns causes water masses to well up from deeper regions. These processes bring deep waters, along with nutrients, into the shelf region at different times of the year. It’s an absolutely natural fertilization process that’s critical for the ecosystem.
The ecosystem also recycles: Phytoplankton are eaten by other organisms that produce waste that contains nutrients, and those become available again for the phytoplankton—much as manure fertilizes a garden.
Some areas of the coastal ocean have been exposed to too many nutrients—which run off from septic systems, lawns, and agriculture, or which are deposited in rain because we put nitrogen into the air. This overnutrition causes the problem of eutrophication, in which rampant algal growth depletes oxygen levels in the water.
What has prevented you from obtaining a clear picture of this dynamic ecosystem?
Answers have eluded us because we had to go out in boats, take single water samples, look at them through the microscope, and meticulously identify each cell—and then you have just one sample. The show-stopper was not having continuous observations of ongoing changes.
What’s really exciting about our new technology is that we can now watch and record the community changing along with the environment, at the same time, in a natural system. There’s still a big puzzle to tease out, because many factors contribute to the changes we see. Now, we can get this fabulous information we need to solve the puzzle.
How do these instruments work?
For many years, my lab group has collaborated closely with Rob Olson’s group at WHOI to develop a submersible instrument we call the FlowCytobot to count and identify tiny phytoplankton in the water. It’s a laser-based system that “sees” single cells—on the basis of red light that the chlorophyll in phytoplankton emits when it’s exposed to blue or green light.
FlowCytobot has been operating almost continuously at the Martha’s Vineyard Coastal Observatory since 2003, and it works great. But we discovered toward the end of 2003 that we had a big problem.
Phytoplankton are diverse, from species too small to see with microscopes to others visible to the eye. We designed the FlowCytobot specifically to look at the smallest phytoplankton out there. But the mixture of plankton species changes during the year. By fall, the ecosystem is dominated by phytoplankton called diatoms, many of which were too big for our instrument to measure.
These population changes are as dramatic as succession on land, where over decades marsh changes to grassland and then to forest. In plankton communities, such dramatic succession happens every year over a few months. It’s critical that we understand what causes these shifts, because if something changes so that the winter diatom blooms don’t occur, for example, that could have serious implications for the rest of the ecosystem.
So could you adjust FlowCytobot to also identify diatoms?
It turns out that it’s not possible to make one instrument that does a good job measuring both the smallest and largest phytoplankton. For diatoms, we needed a way to count cells in larger volumes of water and to take pictures through a microscope, along with the red fluorescence measurements.
We were experimenting with this dual ability in the lab, and colleagues would ask when we were going to build it into a submersible instrument. But it requires so much power, and generates so much data, we at first didn’t think we could put it under water. The idea that you could have an autonomous underwater microscope, and actually run it continuously—we just laughed. But after our experience working at MVCO, it occurred to us that it wasn’t that farfetched. We had had success with the first FlowCytobot, and we knew we had power available. Within a year, we came around to realizing we could do this.
So now there are two instruments, and they work together. The original FlowCytobot counts and measures fluorescence of small cells; then the Imaging FlowCytobot does the same thing for large diatoms, and also takes microscope photographs for identification.
How did you solve the power and data problems?
There’s no doubt in my mind that we wouldn’t have gone down the path of building the imager if we didn’t have the Martha’s Vineyard Coastal Observatory. We can plug our instrument into its seafloor node, which is cabled to shore. The cables provide essentially unlimited, continuous power- and data-transmission capabilities.
FlowCytobot and Imaging FlowCytobot at MVCO will let us look at the entire community from the smallest cells to the largest. If everything works—and we’re crossing our fingers!— we should get data that capture this dramatic shift from the late-summer, small-cell “field of grasses” to this bloom of large organisms in the fall—the “trees.” It will be really interesting to see how that shapes up.
Over the next 10 to 20 years, we envision employing instruments for continuous, long-term monitoring of plankton, nutrients, and oceanographic conditions at a network of several coastal observatories spanning the U.S. East Coast. Then, scientists from many oceanographic disciplines—biology, physical oceanography, and chemistry— can begin to piece together the bigger picture, over time and space. We’ll be able to see the effects of climate change on the ecosystem, for example, or how changes in one region affect other coastal areas.
We’ve been surprised at how difficult it has been to get federal funding for instrument-development work, but support from the WHOI Coastal Ocean Institute and the WHOI Access to the Sea program is helping us install our instruments at MVCO this summer. We think that a successful proof-of-concept deployment will make us poised to be competitive to solicit future funding from national agencies.
What sorts of applications might this research have?
We are very interested in the basic question of what regulates phytoplankton communities. For example, the timing and occurrence of the winter plankton blooms are very important for other species that rely on them as a food source—fish and invertebrates. If the bloom fails one winter, then you may get low survival of fish. We’re all hopeful that, down the road, the kind of basic information we are collecting will allow us to do a better job of managing fisheries and other coastal resources.
First, we need to have a better understanding of the ecosystem’s natural variations. Only then will we be able to be smarter about how to manage human activities in the coastal ocean.
Slideshow
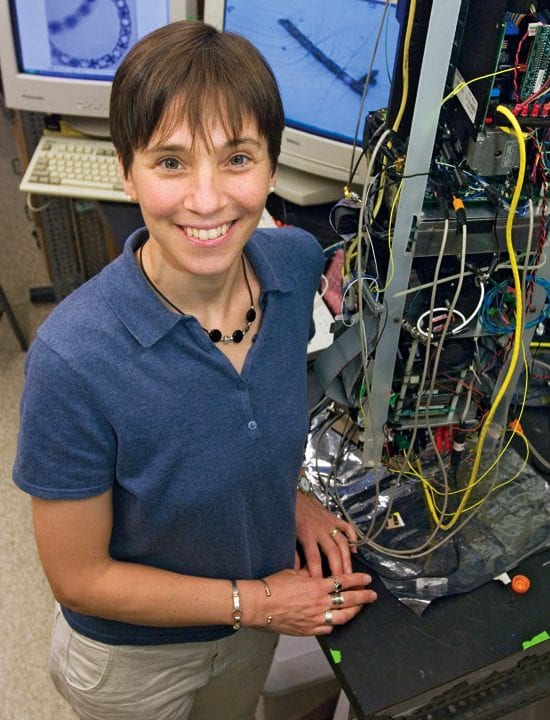
Slideshow
- WHOI biologist Heidi Sosik uses a new robotic instrument to study the tiny plantlike cells that create the food for virtually all ocean life. "If we want the tools to look at these organisms," she says, "we have to invent and make them ourselves. You can?t buy them anywhere." (Photo by Tom Kleindinst, Woods Hole Oceanographic Institution)
- To gather information about coastal ecosystems, WHOI biologist Heidi Sosik traditionally went out in boats, took single water samples, and preserved them to identify phytoplankton cells later in her lab. It was a painstaking process and only provided data about about one place at one time in the coastal ocean. Her new instrument, Imaging FlowCytobot, continously sends images to shore, so she can observe microscopic organisms in real time in their changing environment. (Photo courtesy of Heidi Sosik, WHOI)
- Eucampia, a chain-forming diatom
- Thalassionema, a chain diatom
- Chaetoceros, a diatom common in northeastern U.S. waters
- Thalassiosira, a chain-forming diatom
- Pleurosigma, a single-celled diatom found in costal northeast waters
- The diatom Licmophora, found in coastal waters off Woods Hole, Mass.
Related Articles
- Are warming Alaskan Arctic waters a new toxic algal hotspot?
- The Living Breathing Ocean
- Forecasting Where Ocean Life Thrives
- PlankZooka & SUPR-REMUS
- Illuminating an Unexplored Undersea Universe
- Specks in the Spectrometer
- Setting a Watchman for Harmful Algal Blooms
- Short-circuiting the Biological Pump
- A Telescope to Peer into the Vast Ocean