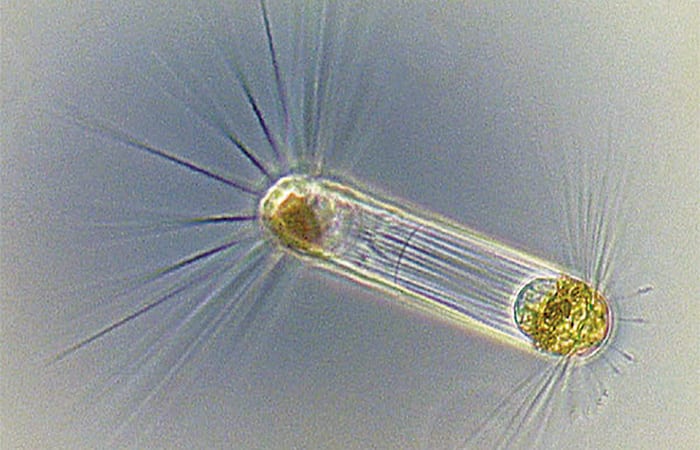
A Day in the Life of a Phytoplankter
A conversation with WHOI biologist Sam Laney
Earth’s vast oceans teem with innumerable microscopic plants that make the fertility and abundance of the United States’ Grain Belt look like, well, a drop in the ocean. These tiny phytoplankton are essential to life on Earth, says biologist Sam Laney.
“Just like plants on land, phytoplankton do photosynthesis: They suck up carbon dioxide and exhaust oxygen. About half of the oxygen on the planet comes from phytoplankton, so every other breath you take is phytoplankton air. In fact, Earth didn’t originally have oxygen in its atmosphere; the evolution of phytoplankton is responsible for there being oxygen for us in the first place.
“Moreover, phytoplankton are the base of marine food webs. Tuna fish or squid ultimately exist because earlier in the food chain, somebody ate some phytoplankton.”
But Laney, a scientist at Wood Hole Oceanographic Institution, has his own individual perspective.
“Most oceanographers think about phytoplankton as a crop or population—you know, a big biomass of plants. They think of them as ‘conduits for geochemistry’— which is fine. But they’re individuals, too. I’m interested in them as individual beings, not just as a collection of millions of cells.”
“So, what is life like for a phytoplankter? If you were a phytoplankton cell, how would you see your environment? What things are important to you? What sorts of situations would you experience over your short lifespan, and what types of things can you do in response?”
How do you explore the lives of individual cells in the ocean?
That’s always been one of the real challenges of phytoplankton ecology: You can look under a microscope and see a cell. But you can’t track it over its lifespan or through its history. Terrestrial ecologists have it easy—they can tag a lion. We oceanographers cannot tag an individual phytoplankton cell (a phytoplankter) and follow it around in the ocean. It’s hard to see the world the way a phytoplankter does.
I try to get around this problem by looking at things a phytoplankton cell can change about itself within a day or two, because that would be its lifespan. Because a population is just a bunch of individuals. If I can tell you how a single diatom—a type of phytoplankton—can respond to a passing cloud, then I can tell you how diatom populations respond to passing clouds.
How does a phytoplankter’s life story begin?
Well, it’s born, but then again it’s not really “born”— a cell and its sister are formed when their parent cell divides in two. Each daughter’s job is to survive long enough to itself reproduce. The name of the game is absorbing enough nutrients and energy to grow large enough that you can divide—and to do that quickly, because somebody is trying to eat you.
While people can eat burgers or mac ‘n’ cheese and get both energy and nutrition out of the one meal, phytoplankton have to get theirs in two separate servings. They get energy from absorbing sunlight, but they have to absorb dissolved nutrients to get their nutrition. In the open ocean, the nutrients near the surface often well up from deeper water. So nutrients are coming from below, but the sunlight is coming from above. If you’re a phytoplankton cell and you’re up too high in the water column, you get lots of light but no nutrients, and if you’re down too low, you get plenty of nutrients but not a lot of light. The best place for phytoplankton to live is in a sweet spot in the middle. That’s one way a phytoplankter sees the world: It’s looking in two directions for the two important things that it needs.
Sounds like an uncertain existence.
Talk about extreme environments! If you’re a tree that lives for years, you can bank on having pretty decent light levels over most of your life. But if you’re a phytoplankton that lives only a few days you might have decent light one day, but then a current pushes you down into the water column so that tomorrow you’ve got little to no light. What do you do if your daily reality is: Will there be light tomorrow?
Passing clouds can also cause fluctuations in sunlight. Over the ocean clouds are often intermittent, so a phytoplankter experiences dim light, then bright light, then dim again, and so on. When the sun rises in the morning, how does a phytoplankter plan ahead? Will it be a uniformly cloudy, or sunny, or a bright-cloudy-bright-cloudy day? It makes a big difference to a phytoplankter. It has to be very flexible in its responses to changing light conditions.
How do phytoplankton harness light energy?
Photosynthesis starts with absorbing sunlight, which is done by chlorophyll and other colored pigments. Chlorophyll is found inside structures called chloroplasts where the individual chlorophyll molecules are packaged into little bundles called “reaction centers.” The job of a reaction center is to absorb a photon of light and shovel that energy into useful energy-storing or electron-storing molecules such as ATP (adenosine triphosphate) and NADPH (nicotinamide adenine dinucleotide phosphate).The cell uses ATP and NADPH in reactions that make compounds the cell needs: the sugars, lipids, amino acids, and DNA. This energy can also go to repairing cell damage, providing energy for swimming if you can swim, and of course for reproduction when it comes time.
It sounds a little like an assembly line in a factory.
Yes, keeping a smooth flow of energy through its reaction centers is really important to plants. Once the reaction center has processed one photon, it’s ready for the next one. It’s just “one photon at a time, one photon at a time …”. But if it has to wait too long between photons, the assembly line is sitting idle. That’s inefficient.
Chlorophyll works like this: When a chlorophyll molecule absorbs a photon, it rings like a bell—it starts resonating. But a chlorophyll can only deal with a certain amount of energy at a time. If it gets two photons’ worth of energy at once, it can break. This is the other reason why plants do as much as they can to keep a smooth flow of energy through all the reaction centers. You don’t want your assembly line idle, but you also don’t want it breaking down when light levels suddenly increase.
What can a phytoplankter do to respond to changing light conditions?
That’s something I’m investigating right now. If a cell experiences lower light it can make more chlorophyll, but that takes many hours and costs a lot of energy. You can’t use that response to adjust to rapid changes in sunlight caused by intermittent clouds, for example. So you need other, faster responses to keep feeding chlorophyll just the right amount of energy: enough so that they’re not idle, but not so much that they get overexcited and break.
To accomplish this, phytoplankton have evolved other types of special pigments, including ones called xanthophylls. In land plants, these are the yellow-colored pigments that remain in leaves in the fall after the green chlorophyll has gone away.
Some of these xanthophylls help phytoplankton absorb more light, but others help them get rid of excess light, acting like sunscreen. Some xanthophylls actually do both and can quickly shift from being a helper pigment to being a protective pigment. Shifts in light ultimately cause tiny shifts in the structure of the xanthophyll molecules. Those slight structural shifts make them behave differently enough so that they either feed light to chlorophyll, or shunt light away, keeping a smooth flow of light energy through the reaction centers.
I’ve done experiments in the lab to try to mimic some of these light level situations to see just how fast and how strong these rapid responses can be. These xanthophyll pigments can flip-flop within minutes to convert from helper into protector pigments. That’s a pretty amazing little trick for a microbe.
I’ve also looked at photosynthetic responses to natural cloud variability in the actual ocean off Hawaii, where it’s not always as sunny as people might think. Most days have some cloud cover, and you might get one cloud-free day every ten or so. People had thought that these pigments flip-flopping back and forth don’t really boost photosynthetic performance much in the real world. But from what I see, I think that maybe it can change phytoplankton’s overall efficiency by 25 percent—which is nothing to sneeze at.
That’s not a trivial amount.
Yeah, exactly—would you take a 25 percent pay cut? That’s what plants are doing when they’re making these photosynthetic adjustments. They are trying to optimize their earnings—how much of the external light field can they turn into useful biological energy?
A 25-percent enhancement over 30 minutes might be enough to matter ecologically—it could make the difference between being able to reproduce today or having to wait until tomorrow to divide. And while you’re standing by overnight, waiting for the sun to come up, you are prey!
What are some other reasons to study phytoplankton as individuals?
We tend to assume that when single-celled organisms divide asexually, they produce two identical daughter cells. Scientists have known for a while that when diatoms divide, they produce one daughter cell that is slightly smaller than its sister.
So I’ve been looking into this: Is there any difference other than size between two diatom daughter cells, and if so, which daughter cell does better? For organisms that give birth to twins, there are a lot of studies about whether the older or the younger twin does better. These are obvious questions to ask about puppies or monkeys or people. Sometimes it surprises me how we’ve gotten this far without asking these same types of questions about phytoplankton.
How did you investigate this and what have you found?
I did an experiment with my WHOI colleagues Rob Olson and Heidi Sosik where I made time-lapse movies of diatom cells dividing generation after generation. I wrote software to keep track of individual cells in these movies.
We just published our study in the September 2012 issue of Limnology and Oceanography, and we showed that the smaller of the two diatom daughters typically inherits more of its parent’s cellular material (or cytoplasm), and that they subsequently grow faster and divide sooner than their larger sisters. It looks like diatoms “favor” the younger daughters somehow.
This is the first time this has been shown in diatoms—that the parent cell actually favors one daughter over its sister. Other studies suggest it’s true in bacteria as well, and so maybe this type of favoritism is a more universal feature in microbes. That could have significant ecological implications.
How did you arrive at this area of research?
I’ve got an engineering degree and a biology degree. I used to think that the engineering degree was useful because it allowed me to build things—which is true. But now I’m beginning to think that the engineering degree is valuable because it allows me to apply “engineering-style thinking” to biological systems.
By “engineering-style thinking,” do you mean thinking about phytoplankton cells as machines?
Exactly! You can look at a machine in a physical sense, with gears and a motor and wires. A phytoplankton cell is like that: There’s stuff inside that does certain jobs, and these things are connected to one another, so that the whole cell acts as a coordinated gadget. A mechanical engineer will get it when you describe a phytoplankton cell in this way.
But you can also look at machines in a more abstract sense, a coordinated network of individual items linked together that, as a whole, do a certain thing—in the case of phytoplankton, to grow and reproduce. This might be the way it’s seen by operations engineers who do factory design and care about the flow of things through a large complex system.
For instance, think about how you might plan for a factory that makes iPads: You have to get different raw materials, and then you have to process these through a factory so that iPads come out the other end. You need to optimize the flow so there are no bottlenecks, so that everything is working smoothly from where the ore is mined to where you buy your iPad in Boston.
When I first started thinking of phytoplankton from an engineering perspective, I thought about them as little computers, asking questions such as: What’s their algorithm for dealing with changes in life? Now it’s more like phytoplankton as “dynamical systems,” which is how an operations engineer might think of this problem of keeping light energy flowing smoothly through a cell.
Describe this dynamical systems approach.
Presumably people at Apple have figured out what happens if they can’t get a given rare metal from a certain source. They build their systems to be robust, so they don’t end up with an idle assembly line and an iPad shortage if a supplier falls through. They have sophisticated software at their disposal to tackle dynamicalquestions such as: “How does a change in the availability of a critical part affect overall production rates? How do you plan for the future when something critical to you might not be always available? That’s a tough mathematical problem.
The mathematical tools that engineers have developed to tackle these problems can now be used by people like me who aren’t operations engineers. In my case, it’s the flow of light through a plant, and what happens if the flow of light is disrupted.
It’s pretty phenomenal, the complexity of the phytoplankton organism, given how supposedly simple it is. It’s not a tree, it’s not a Venus flytrap, it’s a microbe. It doesn’t look complicated compared to bigger plants, but it doesn’t take much for a very simple system to display complex behavior.
You have a philosophical approach.
That’s because I spent the first two years of college studying political philosophy!
Do you recommend that?
Yep! All science students should study a little philosophy, especially the philosophy of science. A lot of people know how to pose a scientific question and address it, but that doesn’t necessarily mean they’ve got a big picture or appreciate the limits to knowledge. Anybody who has studied the philosophy of science will tell you that you can never prove anything; you can at best only disprove something.
Having that liberal arts background helped me perk my ears up to these types of ideas. For me, science isn’t for answering questions; my job is to make questions!
As a scientist you’re on the frontier of knowledge. There is nothing ahead of you but empty space, until you start putting ideas into it. Some of the best scientists are the ones who are comfortable looking into the black, where nothing is known, and realizing how much nothing is known, and then still being willing to take that step forward.
This work was supported by a NASA New Investigator Award, a WHOI Ocean Life Institute Postdoctoral Scholarship, and the WHOI Penzance Fund.
Slideshow
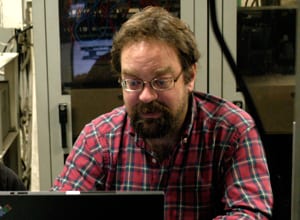
Slideshow
- With backgrounds in engineering, biology, and philosophy, WHOI scientist Sam Laney has a unique perspective on his research. Laney studies phytoplankton, the abundant microscopic plant life at the base of the ocean's food webs. He wants to know what life is like for phytoplankton cells as individuals, and how they adapt to rapidly changing conditions. (Photo by Carin Ashjian, Woods Hole Oceanographic Institution)
Single-celled phytoplankton carry out photosynthesis within specialized organelles called chloroplasts. Like factories, the chloroplasts' photosynthetic machinery requires raw materials and energy—sunlight—to operate. Cells living in the ocean must rapidly adapt to varying light levels caused by intermittent cloudy and bright conditions.
(Illustration by Amy Caracappa-Qubeck and Katherine Joyce, Woods Hole Oceanographic Institution)
» pdf version of illustration- Scientist Sam Laney and research assistant Emily Peacock studied the types and abundance of phytoplankton in Arctic water on a 2010 cruise aboard the U.S. icebreaker Healy. An "ice liberty" afforded a chance to get a short distance away from the ship for a brief time. (Photo by Karen Romano Young)
- On a recent research cruise to the Artic, WHOI research assistant Emily Peacock, a member of Sam Laney's lab, operated an Imaging FlowCytobot, an instrument that images and identifies individual phytoplankton cells in ocean water. Sam Laney adapted the instrument, first developed by WHOI biologists Heidi Sosik and Rob Olson, for use at sea and to investigate phytoplankton populations in polar waters. (Photo by Karen Romano Young)
- Diatoms, a type of phytoplankton, can be single cells or form chains of cells. The seagoing Imaging FlowCytobot took this image of a chain-forming diatom from Arctic waters on a 2010 research cruise. In this diatom species, long spines protruding from the cells hold the cells together as a group. (Image courtesy of Sam Laney, Woods Hole Oceanographic Institution)
- This image, taken on the same Arctic cruise with Sam Laney's seagoing Imaging FlowCytobot instrument, shows chain-forming diatoms in the act of dividing asexually. The pair of cells on the left has recently divided, producing two daughter cells from one parent cell. The cells on the right are still in the process of dividing. The daughter cells are building new cell walls between them, but are not yet fully separated from each other. Daughters differ slightly in size, though it is not obvious in this image. (Image courtesy of Sam Laney, Woods Hole Oceanographic Institution)
- Phytoplankton are the base of ocean food webs, like grass on the prairie. When nutrients and sunlight are plentiful, microscopic phytoplankton cells develop huge population explosions called blooms. Some blooms are so massive that they tint the water and can be seen from space, as in this satellite image of a bloom between New Zealand's North and South Islands. Different colors swirling in the ocean−white, green, and brown−are likely due to different kinds of phytoplankton, including diatoms. (NASA Ocean Color Group)
Related Articles
- Are warming Alaskan Arctic waters a new toxic algal hotspot?
- The Living Breathing Ocean
- Forecasting Where Ocean Life Thrives
- PlankZooka & SUPR-REMUS
- Illuminating an Unexplored Undersea Universe
- Specks in the Spectrometer
- Setting a Watchman for Harmful Algal Blooms
- Short-circuiting the Biological Pump
- A Telescope to Peer into the Vast Ocean