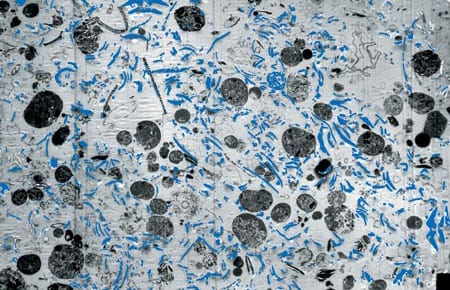
The Rain of Ocean Particles and Earth’s Carbon Cycle
WHOI Phytoplankton photosynthesis has provided Earth’s inhabitants with oxygen since early life began. Without this process the atmosphere would consist of carbon dioxide (CO2) plus a small amount of nitrogen, the atmospheric pressure would be 60 times higher than the air we breathe, and the planet’s air temperatures would hover around 300°C. (Conditions similar to these are found on Earth’s close sibling Venus.
December 1997 — Phytoplankton photosynthesis has provided Earth’s inhabitants with oxygen since early life began. Without this process the atmosphere would consist of carbon dioxide (CO2) plus a small amount of nitrogen, the atmospheric pressure would be 60 times higher than the air we breathe, and the planet’s air temperatures would hover around 300°C. (Conditions similar to these are found on Earth’s close sibling Venus).
As phytoplankton grow through the process of photosynthesis, they fix CO2 in their cells as organic carbon and thus absorb atmospheric CO2 into the upper ocean layers. Animal plankton graze the phytoplankton, employing most of the organic carbon as their energy source and oxidizing the rest of it back to CO2, which eventually returns to the atmosphere. A small portion of this photosynthetic carbon escapes the oxidation process by settling, or “sedimenting,” through the water column in particles, which are often called “marine snow” and include pelletized feces of small animal plankton. CO2 carbon is also transported to the deep ocean in another way: Some plankton, such as coccolithophorids, planktonic foraminifera, and pteropods produce beautiful calcite and aragonite shells or tests that sink toward the seafloor when the organisms die.
Once the organic carbon and calcium carbonate particles reach the ocean interior at a depth of a few kilometers, they are “stored” there and will not return to the atmosphere for a relatively long period of time. This complex carbon-transporting ocean process, often called the “biological pump,” is a critical mechanism in preventing what we now know as the “greenhouse effect,” the collection of gases in the atmosphere that hinders upward transport of heat.
Understanding of Earth’s carbon cycle is one of humankind’s great scientific questions. Sediment traps are an important tool for studying the spatial and temporal variability of sinking particles (and carbon) in the ocean. The idea behind these devices is very simple: Vertically settling particles are collected at a specific area during a specific time period by providing a stable collection area at a depth along a mooring. The collected particles are then recovered and weighed, and the vertical flux of particles can be calculated as weight or volume per unit area in a unit of time—milligrams per square meter per day. Because the export flux of carbon is usually highly seasonal and often episodic, a short-term measurement produces data that is only useful for limited special purposes. It is therefore critical to collect sediment in time series for at least a year. Though this is not as technologically easy as this simple description may sound, the time-series sediment trap array method, together with multidisciplinary ocean measurements, has recently brought large leaps in the understanding of basinwide dynamics of the biological pump in relation to such global oceanographic phenomena as El Niño and the Asian monsoons. Sediment trap experiments have come to be one of the principal methods for understanding global CO2 cycles in the ocean.
We now have 15 years of time-series, sediment-trap data collected from the interior of the world’s open oceans through the collaborative effort of an international group of scientists, including the WHOI PARFLUX group. We are finally beginning to understand the pattern of basin-to-basin export-flux variability and to make intelligent estimates of the flux of CO2 carbon in particulate matter to the ocean’s interior. A sediment trap collects not only carbon products but also other kinds of particles: For example, we have found that the export flux of biogenic silica produced by plankton with siliceous frustules and tests provides important information for understanding another type of basin-scale biological pump (see Arabian Sea article).
By integrating sediment trap based measurements (figure above), we estimate the global flux of carbon to the ocean’s interior at 0.8 gigaton, nearly one billion tons, per year—0.35 gigaton in organic carbon and 0.44 gigaton in inorganic carbon (calcium carbonate). The global calcium in carbonate and silicon in biogenic opal export fluxes are 2.9 and 1.4 gigatons per year, respectively. Interannual fluctuation of global export production is unknown, as we are far from understanding the interannual changes in overall primary production.
Our 15-year export flux data set reveals distinct “biogeochemical regions” where the biological pump operates in two significantly different modes known as “Carbonate Ocean” and “Silica (Opal) Ocean” (see figure above right). A Carbonate Ocean is defined as the condition in which sinking particles deliver more carbonate than silica to the ocean interior, as well as more inorganic than organic carbon. In a Silica Ocean, these ratios are reversed. To date, these conditions are only observational, and we are anxious to determine the reasons for this partition in the ocean. Global distribution of dissolved silica and other essential nutrients, which is controlled by very large scale ocean circulation, might be the deciding factor in this partition. The majority of present seas are Carbonate Oceans while Silica Oceans account for less than 20 percent of the world ocean. The export flux of organic carbon in a Silica Ocean is usually higher than that in a Carbonate Ocean because their biological pumps function differently: Photosynthesis of diatoms, whose shells are largely composed of silica, is the mechanism for primary production in a Silica Ocean, and photosynthesis of coccolithophorids, whose shells are largely composed of calcium carbonate, is the primary producer in a Carbonate Ocean.
At present, one of the most typical Silica Oceans is the sub-Arctic Pacific north of 45°N, an area that includes the Bering Sea, the Sea of Okhotsk, and the northern East Sea (Japan Sea). By contrast, most of the North Atlantic is a Carbonate Ocean. Nutrient availability is the major oceanographic difference between these seas. Layers rich with dissolved nutrients underlie the sub-Arctic Pacific at about 600 meters; at 2,000 meters the dissolved silica maximum is reached. In contrast, the amount of silica in the northern North Atlantic is an order of magnitude less than in the sub-Arctic Pacific.
In the northern North Atlantic, upper ocean waters sink due to thermal exchange and are replaced with nutrient-depleted surface waters supplied from the south. This results in a negative silica mass balance in the upper layers in this area and allows coccolithophorids to take over the niche from the diatoms because coccolitho-phorids need no dissolved silica to grow. On the other hand, the upper layers of the sub-Arctic Pacific are enriched by the upwelling of dissolved silica. A large flux of biogenic opal particles is supplied to the relatively shallow but vast shelves and slopes in the Sea of Okhotsk and the Bering Sea and is recycled to the upper waters, generating the positive feedback loop of a “silica trap.” Thus the ecosystem is essentially occupied by diatoms, and relatively scarce coccolithophorids appear only when the surface ocean is temporarily depleted of silica at the end of the export-flux bloom.
The biological pump operating in a Silica Ocean removes CO2 carbon from the upper to the deep ocean in the form of particulate organic carbon on a short time scale of a few to several weeks (see Arabian Sea article). The biological pump in a Carbonate Ocean also removes carbon from the atmosphere and upper oceans in the form of organic carbon. However, when a molecule of calcium carbonate is formed in the upper ocean as part of a coccolith or planktonic foraminifer test, a molecule of CO2 is also formed by this chemical reaction. Therefore, there is no net removal of CO2 carbon from the upper oceans by the settling of inorganic carbon as calcium carbonate. Secretion of calcium carbonate in the upper ocean by organisms reduces alkalinity, resulting in an environment less capable of absorbing atmospheric CO2. Therefore, a Carbonate Ocean does not play a large role in removal of atmospheric CO2. The significance of the biological pump in a Carbonate Ocean is principally concerned with the long term CO2 balance in Earth’s atmosphere (see figure above).
As soon as they leave the upper ocean where they are produced, almost all of the coccoliths and foraminifera settle to the deep ocean floor within a short time. There the oxidation of organic matter that begins in the upper ocean causes an increasingly acidic condition, which results in dissolution of the coccoliths and foraminifera. Indeed, researchers find it strange to retrieve a large quantity of perfectly preserved coccoliths and foraminifera tests from a sediment trap that has been moored above a deep Pacific seafloor that consists of nothing but red clay and a few fragmented calcareous remains.
The deep ocean water, whose increased alkalinity is due to the dissolution of calcium carbonate, moves in such deep currents as the thermohaline driven circulation that runs south from the northern North Atlantic, proceeds as a bottom current through the Indian Ocean, and eventually upwells in the northern North Pacific (See Oceanus Vol. 39, No. 2). This mass of formerly deep water that has moved to the surface is thought to be a major absorber of atmospheric CO2. This mechanism is often called the Alkalinity Pump (different from the Biological Pump). Note that this process is too slow to affect the CO2 cycle of today’s Earth—it takes several centuries for the ocean’s deep-water circulation system to complete a turnover. Our present climate is greatly dependent on foraminifera that lived, died, and dissolved when Vikings roamed the ocean! In other words, calcium carbonate that sinks to the deep corrosive ocean is a “savings deposit” regulating future greenhouse effects of planet Earth well into the next millennium.
Major funding sources for Sus Honjo’s sediment trap work have been the National Science Foundation and the Office of Naval Research.
During his long research career at WHOI, Sus Honjo has concentrated on understanding the processes and rates of ocean sedimentation and the removal of carbon in the form of carbon dioxide from the atmosphere to its deep ocean “sink.” He recalls trembling with excitement when he looked into a microscope aboard R/V Knorr to examine the first sample collected by a deep ocean sediment trap in the central North Atlantic: Abundant fecal pellets produced by surface organisms proved his hypothesis that the majority of ocean sediment is delivered to the deep seafloor by large, rapidly settling aggregates. His global research efforts to link upper and deep ocean processes bridge marine geology, ocean chemistry, and biology and enhance understanding of the biogeochemistry of the ocean. As we prepared this issue, he was sending cruise reports from the southern ocean near Antarctica where he was leading a team of “trappers” and seafloor sediment research groups from WHOI and other US institutions in rough seas aboard NSF’s ice breaker/research vessel Nathaniel B. Palmer.
Slideshow
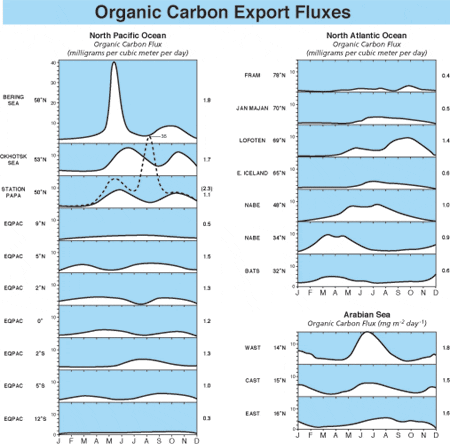
Slideshow
- Integration of sediment trap based measurements from a variety of locations allows investigators to estimate the global flux of carbon to the oceanA?s interior. (Jack Cook)
- Conditions that influence removal of carbon-dioxide carbon from atmosphere to deep ocean are illustrated here and discussed in the article. (Jack Cook (thanks to Dan McCorkle))
- Fecal pellets are about the size of a comma (,).
- Transmision electron microscope image (enlarged some 3,000 times) of a section of a fecal pellet collected by a sediment trap in the North Atlantic. It includes undigested, phytoplankton cell organelles, including chloroplasts. The blue (artificially colored) areas represent remains of coccoliths that were eaten by a zoolankter and passed unaffected through the gut. Coccoliths are composed of nearly pure calcite, the heaviest mineral produced by marine organisms, which makes a fecal pellet heavy enough to settle rapidly through the water column. (Susumu Honjo)
- Organic carbon flux measured over at least one year with time-series sediment traps in the Northern Hemisphere in grams per square meter per year. This figure integrates data derived from sediment traps around the world, including WHOI PARFLUX results. Note the huge export flux in the Arabian Sea and relatively large annual flux in the northwestern Pacific. The "biological pump" works efficiently in these areas compared to the mid ocean.
- The ratio of silicon to calcium in organism-assimilated particles collected by deep ocean sediment traps is an indicator of the rate of removal of carbon-dioxide carbon from the upper ocean to the deep-ocean "sink." This ratio indicates there are two types of ocean areas depending on which element is dominant (see figure 2 above).