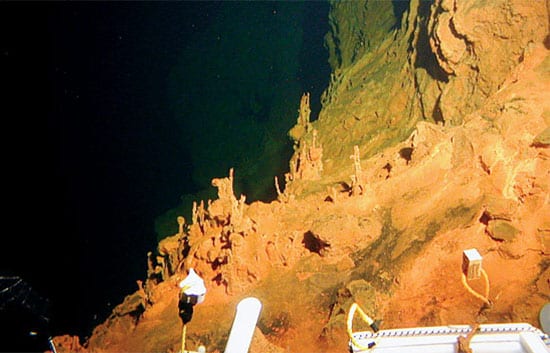
Living Large in Microscopic Nooks
Newly discovered deep-sea microbes rearrange thinking on the evolution of the Earth? and life on it
Between a rock and a hard place is the proverbial worst spot for people to find themselves in. But for certain deep-sea microbes, it’s the place to be. In 2000, to our surprise, we found that microscopic nooks and pits within volcanic seafloor rocks harbor abundant colonies of previously unidentified microbes.
These microbes are different from other microorganisms living in the sunless depths. They do not obtain the energy they need to grow and multiply by metabolizing chemicals dissolved in seawater or in hydrothermal fluids venting from the seafloor. Instead, these newly discovered microbes are living directly off minerals in solid seafloor rocks.
The microbes are oxidizing iron in the rocks, chemically altering the rocks, and harnessing the energy produced by this chemical reaction to live. Their discovery has raised a slew of intriguing questions:
- Does our planet sustain abundant and ubiquitous populations of these microbes?
- Do they play a pivotal role in chemically altering Earth’s crust?
- Were they pioneering life forms on an early Earth, which was largely devoid of oxygen but full of iron?
- Do they exist on other iron-rich, oxygen-poor planetary bodies such as Mars?
These previously inconspicuous microorganisms may turn out to have starring roles in shaping the evolution of life on Earth and other planets, and shaping the evolution of the planet itself.
So why didn’t we notice them before? Beyond the inherent difficulties and expense of searching for microorganisms at the bottom of the ocean, the answer is that we hadn’t really looked for them before. But now these easy-to-overlook microbes have become hard to ignore.
Pumping iron on the seafloor
More and more, we are learning how life on the Earth and the Earth itself—biology and geology—are intimately intertwined and evolve together. Microbes are ubiquitous catalytic agents, sparking chemical reactions that alter the physical and chemical properties of their surroundings. Beyond our scope of vision, their cumulative metabolic activities play a fundamental role in shaping and regulating our environment. (Our world would be completely different, for example, if microorganisms did not continuously decompose organic matter and transform it back into inorganic material.)
A new field has arisen called geomicrobiology. Scientists are now taking a closer look at many unexplored regions of our planet, and other planets, searching for populations of unknown microbes that may play major roles in cycling chemicals through planetary systems.
In geomicrobiology, the borders between rocks and living things are not so ironclad. Many rocks are, however, and the microbes we found steal electrons from iron atoms in the rock, changing them from ferrous (Fe+2) to ferric (Fe+3). With the energy produced by this chemical reaction, they convert carbon dioxide (from seawater) into organic matter—much the way plants and plankton use solar energy and photosynthesis to accomplish the same.
Microscopic, but mighty
Iron is one of the most abundant and reactive elements in the environment near Earth’s surface, so the discovery of iron-oxidizing microbes raises the potential that massive communities of them may exist on Earth. If so, they could continually extract huge amounts of carbon dioxide from seawater and microscopically exert a huge influence on ocean chemistry over geologic time.
Does this large-scale drawdown of carbon dioxide from seawater help the oceans absorb carbon dioxide, a critical greenhouse gas, from the atmosphere? If so, it would revise our understanding of how carbon cycles through the planetary system—perhaps giving iron-oxidizing microbes an important, previously unknown role in the evolution of Earth’s climate.
In their own way, the rise of microscopic photosynthetic plants caused one of the most devastating, permanent alterations in all of Earth’s history. They changed the chemical composition of the near-surface environment that all life depended on, by simply pumping oxygen into Earth’s atmosphere.
Before then, neither the atmosphere nor the oceans contained much oxygen, but the oceans were filled with iron-rich rocks and tons of dissolved iron. In such an iron-rich, oxygen-poor environment, iron-oxidizing microbes may have been dominant, pioneering life forms—a concept that compels us to reassess our thinking about the evolution of life on early Earth.
The existence of iron-oxidizing microbes also redirects our search for life elsewhere in the universe. Similar microbes could have thrived, or still thrive, in other iron-rich, oxygen-poor locales—such as Mars, with its red, iron-rich soil, or on the volcanic seafloor below the ice-covered ocean of Jupiter’s moon, Europa.
A search for unknown life
These unexpected new lines of inquiry began in 2000 when former WHOI Postdoctoral Scholar Tom McCollom and I, with funding from the Mellon Foundation and the National Science Foundation (NSF), joined a research cruise aboard R/V Atlantis off the Oregon coast.
Since the late 1970s, when hydrothermal vents were discovered, scientists have focused on deep-sea chemosynthetic microbes that derive energy from dissolved hydrogen, hydrogen sulfide, and methane emitted from these sites. Though it is easier for microbes to draw energy from chemicals dissolved in seawater, WHOI biologist Carl Wirsen and others had found evidence of sulfur-oxidizing bacteria that used solid minerals as their only source of energy. (See Is Life Thriving Beneath the Seafloor?)
Enormous amounts of sulfur and sulfides are found in vent chimney rocks, in broken chimney rubble on the seafloor, and in fine-grained mineral particles that precipitate and “rain” out of plumes of hydrothermal fluids spewing out of chimneys. We speculated that this little-recognized but potentially large source of chemical energy may sustain important microbial communities, which, in turn, could play pivotal roles in altering the chemistry of seafloor rocks and the ocean itself.
Our goal in 2000 was to try to identify unknown microbes that live off solid minerals and that might be mediating large-scale geochemical changes on Earth.
The perfect niche for microbes
To explore what might be down there, we used the submersible Alvin to place a variety of microbe-free samples of natural seafloor rock back on the seafloor. Our aim was to see what might “grow” on these “blank slates.”
WHOI geochemist Meg Tivey retrieved our experimental samples for us during an Alvin dive two months later (SeeThe Remarkable Diversity of Seafloor Vents). To our surprise, we found that many of the samples had thick burnt-orange coatings of oxidized iron (or “rust”).
Using a scanning electron microscope, we saw that the surfaces of the samples were scarred with abundant pits and pores less than 20 microns (0.0004 inches) deep and wide. In these tiny pits were large accumulations of corkscrew-shaped stalks made of iron oxide, which created the thick rusty coating.
Here’s what we believe is happening: Iron-oxidizing microbes exploit a niche where the chemistry is just right. At first, oxygen-loving microbes move into the pits. They consume the available oxygen, which is not replenished because seawater does not readily flow into the restricted pit areas.
That creates an ideal situation for the iron microbes, which need low-oxygen conditions. The tiny sheltered coves within seafloor rocks contain just enough oxygen from seawater for the iron microbes to respire, but not an overwhelming amount that would oxidize all the iron—without microbial intervention—before the microbes could use it.
As a byproduct of their iron-oxidizing process, the microbes produce bundles of iron-oxide stalks that resemble a little girl’s braids. These stalk accumulations effectively cap the pits, maintaining the iron microbes’ preferred low-oxygen environment and securing their turf.
FeMO—a microbial observatory
The rapid proliferation and sheer abundance of these iron microbes and the quick chemical transformation of the rocks they lived on were eye-opening. Now we have mobilized research that combines biology, chemistry, and geology to explore many intriguing aspects of these iron microbes.
Among the initial questions are: What kinds of iron-oxidizing microbes are out there? How many are there? How are they making a living?
These species have been notoriously difficult to grow in the laboratory and therefore difficult to learn about. But in our lab Dan Rogers and I, along with WHOI biologist Eric Webb and others, have made strides recently to culture and interrogate these elusive microbes, and we have begun to identify various species of microbes and reveal their biochemical machinery and metabolic capabilities.
Toward this end, we have just established “FeMO”—an Iron (Fe)-oxidizing Microbe Observatory—to study these microbes at a site where they are diverse and prolific. It is located at Loihi, an active, submerged volcano, relatively conveniently located only 25 miles southwest of the big island of Hawaii.
To investigate the potential abundance of iron microbes, WHOI geochemist Wolfgang Bach and I analyzed rock samples retrieved from an assortment of holes drilled by the Ocean Drilling Program into the exposed volcanic rock that spreads out on both sides of the mid-ocean ridge mountain chain encircling the globe. We found that older rocks were depleted of Fe+2 and full of Fe+3—exactly what iron-oxidizing microbes use up and leave behind. The finding suggests that mid-ocean ridge flanks represent millions of square miles of fertile habitat for iron microbes.
Life on early Earth and elsewhere
We have also begun to sequence genomes of these microbes, in a project with Mitch Sogin and Ashita Dhillion at the Marine Biological Laboratory in Woods Hole, funded by the National Aeronautics and Space Administration’s Astrobiology Institutes Program (NAI). These microbes are pioneers that probably lived billions of years ago on Earth and may exist on other planetary bodies. Identifying their genes, the enzymes they produce, and the metabolic pathways these enzymes catalyze will reveal an evolutionary heritage that will help us unravel the emergence and development of life on Earth and guide our search for life elsewhere in the universe.
A key to reconstructing the evolution of life on Earth and other planetary bodies lies in the ability of scientists to read the records, or “biosignatures,” that long-dead microbes leave behind in ancient or extraterrestrial rocks. To do that reliably, scientists must be able to distinguish changes caused by microbial activity from those caused by abiotic oxidizing processes such as rusting.
With this goal, scientists in our group, including Bach, Postdoctoral Scholar Olivier Rouxel, and graduate student Cara Santelli, are advancing a range of new approaches to gain understanding of how microorganisms affect the microtextures, isotopic chemistry, and history of the rocks they interact with.
If we can unravel their story, these long-neglected microbes will reveal a profound tale about the co-evolution of Earth and life.
Slideshow
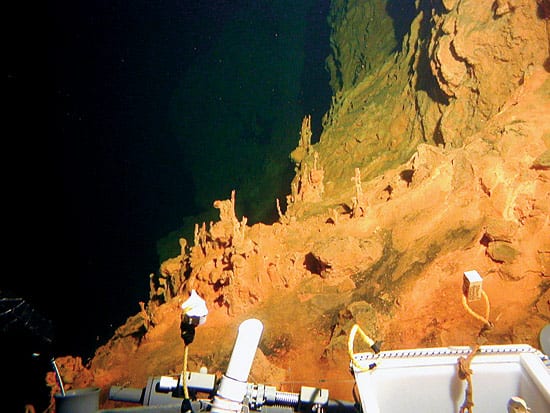
Slideshow
- RUST IN DAVEY JONES' LOCKER—Reddish-orange iron oxide (the same chemical compound we commonly refer to as "rust") coats the seafloor on Loihi Seamount, an active underwater volcano 25 miles off the island of Hawaii. The material is made by an abundance of microbes that live and grow by oxidizing iron directly from solid seafloor rocks. To study these newly discovered microbes, scientists have established FeMO—the Iron (Fe)-oxidizing Microbe Observatory—on Loihi. (Terry Kirby, University of Hawaii.)
- TRANSFORMING ROCK—Rusty-orange iron oxide coats the left side of this sample of seafloor rock, where microbes have oxided iron in the rock. They harness the chemical energy from this reaction to live and grow. The microbes did not progress to the right side of the rock, which remains its normal gray color. (Katrina Edwards)
- A BUCKETFUL OF DATA—WHOI scientists prepared plastic buckets filled with thin, microbe-free samples of natural seafloor rock and placed them back on the seafloor. The experiment sought to find out what might “grow” on these “blank slates.” To their surprise, the scientists found that the samples were quickly colonized by intriguing microbes. (Katrina Edwards)
- An experimental sample of seafloor rock is put back on the seafloor.