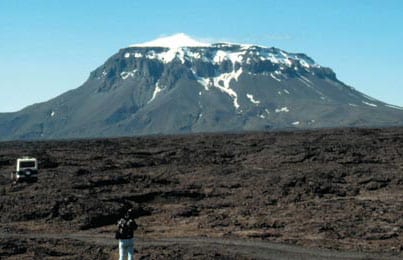
Hitting the Hotspots
New Studies Reveal Critical Interactions Between Hotspots and Mid-Ocean Ridges
1998— The great volcanic mid-ocean ridge system stretches continuously around the globe for 60,000 kilometers, nearly all of it hidden beneath the world’s oceans. In some places, however, mid-ocean ridge volcanoes are so massive that they emerge above sea level to create some of the most spectacular islands on our planet. Iceland, the Azores, and the Galápagos are examples of these “hotspot” islands—so named because they are believed to form above small regions scattered around the earth where unusually hot rocks rise from deep inside the mantle layer.
But hotspots may not be such isolated phenomena. Exciting advances in satellite oceanography, seismology, geochemistry, and geodynamics, along with a treasure trove of new declassified data, are revealing that hotspots appear to have important and far-reaching impacts on a surprisingly large percentage of the global ridge system. Some 44 hotspots have been identified around the globe, and a large number of them are integrally connected to the ridge system (see map below right). Indeed, these hotspots may play a critical role in shaping the seafloor—acting in some cases as strategically positioned supply stations that fuel the lengthy mid-ocean ridges with magma.
Studies of ridge-hotspot interactions received a major boost in 1995 when the US Navy declassified gravity data from its Geosat satellite, which flew from 1985 to 1990. The satellite recorded in unprecedented detail the height of the ocean surface. With accuracy within 5 centimeters, it revealed small bumps and dips created by the gravitational pull of dense underwater mountains and valleys. Researchers often use precise gravity measurements to probe unseen materials below the ocean floor. In places where the seafloor contour has been well-charted by ship surveys, we can employ modeling to remove the gravitational effects of seawater and the seafloor. The leftover signal, called the Bouguer anomaly, reveals information about the rocks beneath the ridges and hotspots. Using this technique, we have detected unusually thick, hot crust and mantle rocks beneath virtually all major hotspots located near ridges, including Iceland and the Azores in the northern Atlantic Ocean, Tristan de Cunha in the southern Atlantic Ocean, the Galápagos and Easter Islands in the Pacific Ocean, and the Marion and Bouvet hotspots in the southwest Indian Ocean.
Beneath the earth’s thin outer skin (called the lithosphere), mantle rocks creep plastically in a layer known as the asthenosphere, where temperatures stay near the rocks’ melting point. Below the mid-ocean ridges, mantle rocks rise to fill the gap between two separating plates. As they ascend, some mantle rocks liquefy to form basaltic magmas, or melts, and the buoyant magmas float to the top of the mantle to form oceanic crust.
By studying the chemical composition of rocks dredged from the ocean floor, researchers have determined that most melts are probably produced at depths of 20 to 80 kilometers and at temperatures of 1,150° to 1,400°C. Below a hotspot, however, geochemical evidence shows that mantle rocks may start melting at greater depths and higher temperatures, giving rise to voluminous lavas and melts that solidify to form shallow ridges such as the Reykjanes Ridge near Iceland, underwater volcanic plateaus such as the vast Kerguelen Plateau in the southern ocean, hotspot islands such as Hawaii, and smaller submerged volcanoes called seamounts.
By measuring the precise travel time of seismic waves passing through the mantle rocks under Iceland, researchers have recently identified a surprisingly narrow cylindrical “root” of anomalously hot rocks extending to at least 400 kilometers beneath Iceland (see figure above right). Below 400 kilometers, seismic data cannot be easily gleaned, but more indirect seismic evidence suggests that this narrow root, which is about the width of Iceland, may be underlain by a region of anomalously hot mantle as deep as 660 kilometers. Theoretical geodynamic models show that such a narrow hotspot root would generate a vigorous source of heat, providing a huge volume of lava to build and feed Iceland’s volcanic landscape. Even more dramatically, this heat source would also cause mantle rocks to migrate laterally in the asthenosphere hundreds of kilometers out from Iceland (see figure below right).
The Geosat data show that in the cases of Iceland and the Azores, a bulge of unusually thick and elevated crust extends from the two hotspots in both directions along the Mid-Atlantic Ridge. This region of thick, hot crust and mantle extends 1,300 kilometers north and south of the Iceland hotspot and some 1,000 kilometers north and south of the Azores hotspot. Together, the two hotspots appear to be feeding a huge supply of magma to nearly the entire northern segment of the Mid-Atlantic Ridge. These hotspots, like others, don’t seem to provide a steady supply of magma, but rather periodic surges. What regulates this episodicity is an intriguing question currently under investigation.
In the far southern Atlantic, a string of hotspots—Tristan, Gough, Discovery and Shona—align with the southern Mid-Atlantic Ridge, and may be contributing magma supplies that maintain the ridge (see map bottom right). Just to the east of the Shona hotspot lies the Bouvet hotspot, whose geological importance may go far beyond creation of the tiny, remote island of Bouvet. Researchers speculate that the hotspot may play a critical role in maintaining the triple junction, where the mid-ocean ridge branches that define the boundaries of the South American, African, and Antarctic plates all intersect. Farther east, the prominence of the Kerguelen, Crozet, and Marion hotspots suggests that ridge-hotspot interactions have helped shape the seafloor of the
southern ocean.
In the western Pacific, our studies show a complex pattern of interaction between the Galápagos hotspot and the Cocos-Nazca Ridge, the boundary between the Cocos and Nazca plates. About 8 million years ago, the ridge and hotspot collided and the hotspot-induced melt flux was estimated to be very robust. But comparisons of older and younger crust in the region reveal that the intensity of the ridge-hotspot interaction has been diminishing as the Cocos-Nazca Ridge gradually moved away from the Galápagos hotspot. Today the two are 200 kilometers apart, and we theorize that the hotspot will lose its impact on the ridge when the two reach a distance of about 500 kilometers apart.
Emerging geophysical and geochemical data indicate significant dissimilarities among hotspots and tell us that the interactions between individual hotspots and ridges have their own peculiar dynamics. While hotspots such as Iceland and Hawaii may have their origin deep in the mantle, others may simply reflect large concentrations of unusual chemical properties in the earth’s shallow mantle. While Iceland’s impact on the ridge extends up to 1,300 kilometers north and south, the Marion hotspot’s effect on the Southwest Indian Ridge diminishes after only 300 kilometers. Magma eruption rates vary among different hotspots, and each hotspot has its own distinguishable geochemical signature, which provides clues to understand how melting occurs at each hotspot. Iceland and the Azores, for example, both show an elevated ratio of stronium 87/stronium 86 isotopes in the basaltic rocks they produce, compared with basalts produced in Mid-Atlantic Ridge regions farther south. However, basalts generated by the Azones hotspot do not exhibit the same elevated helium 3/helium 4 isotopic ratios that the Icelandic basalts do.
Rapidly progressing techniques in geochemistry, as well as in broadband seismology, autonomous underwater geophysics, and geodynamic modeling—combined with the new global geophysical data coverage—should make the coming decade a most exciting time to study the fascinating geological phenomenon of ridge-hotspot interactions.
The National Science Foundation supported the research described. The author is indebted to MIT/WHOI Joint Program graduates Garrett Ito (now at University of Hawaii), Javier Escartin (now at CNRS, Paris, France), and current student Jennifer Georgen for sharing exciting time at sea and ashore in studying mid-ocean ridges and oceanic hotspots.
Jian Lin joined WHOI in 1988 after graduate study at Brown University and research on earthquakes at the US Geological Survey in Menlo Park, California. He divides his travel time between going to sea and investigating quakes in California. His latest expedition was on board the French ship L’Atalante to the Mid-Atlantic Ridge south of the Azores hotspot, where spectacular new underwater volcanoes were discovered.
Slideshow
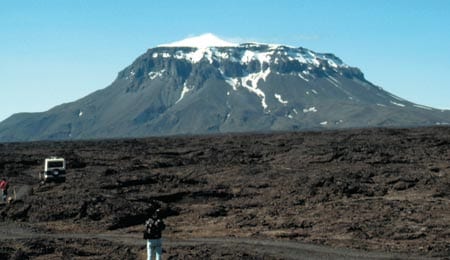
Slideshow
- A hotspot created the island of Iceland and its characteristic volcanic landscape. Hotspots are relatively small regions on the earth where unusually hot rocks rise from deep inside the mantle layer.
- A map of the world's major hotspots shows that many of them are integrally connected to the global mid-ocean ridge system (red lines). Green lines indicate subduction zones, where plates plunge back into the mantle. Not shown is the Jan Mayen hotspot north of Iceland.
- By measuring precise travel times of seismic waves passing through mantle rocks beneath Iceland, scientists have recently identified a surprisingly narrow and deep cylindrical "root" of anomalously hot rocks, extending to a depth of at least 400 kilometers, and probably farther. (From: Wolfe, Bjarnason, VanDecar, and Solomon, 1996)
- Recently declassified satellite gravity data reveal bulges of unusually thick and elevated oceanic crust (red, yellow and green on the map) extending hundreds of kilometers from the Iceland and Azores hotspots. The two hotspots may be feeding huge supplies of magma to nearly the entire northern segment of the Mid-Atlantic Ridge. Map produced by Jennifer Georgen, MIT/WHOI Joint Program, with data from of David Sandwell, Scripps Institution of Oceanography, and Walter Smith, NOAA
- A theoretical geodynamic model shows that a narrow hotspot "root," such as the one recently discovered beneath Iceland, would generate a vigorous source of heat (light colors) and produce a huge volume of magma that would migrate laterally along and across the ridge axes hundreds of kilometers away from the hotspot source.
- Satellite gravity map of the western Indian and southern Atlantic Ocean basins, as revealed by satellite gravity data. A string of hotspots (Tristan, Gough, Discovery, and Shona) aligned with the southern Mid-Atlantic Ridge (MAR) may be contributing magma supplies that maintain the ridge. The Bouvet hotspot may play a critical role in maintaining the triple junction, where three mid-ocean ridge branches and the three boundaries (white lines) separating the South American, Antarctic, and African plates all intersect. Farther east, the Kerguelen, Crozet, and Marion hotspots may have extensively shaped the seafloor of the southern ocean. SEIR is the Southeast Indian Ridge, CIR is the Central Indian Ridge, and SWIR is the Southwest Indian Ridge. Map produced by Jennifer Georgen, MIT/WHOI Joint Program, with data from David Sandwell, Scripps Institution of Oceanography, and Walter Smith, NOAA.