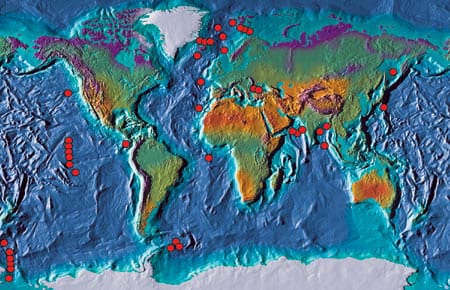
Ground-Truthing the Paleoclimate Record
Sediment Trap Observations Aid Paeoceanographers
December 1997 — The geological record contains a wealth of information about Earth’s past environmental conditions. During its long geological history the planet has experienced changes in climate that are much larger than those recorded during human history; these environmental conditions range from periods when large ice sheets covered much of the northern hemisphere, as recently as 20,000 years ago, to past atmospheric concentrations of greenhouse gases that warmed Earth’s polar regions enough to melt all of the ice caps 50 million years ago. Since human civilization has developed during a fairly short period of unusually mild and stable climate, humans have yet to experience the full range of variability that the planet’s natural systems impose. Thus, the geological record has become an extremely important archive for understanding the range of natural variability in climate, the processes that cause climate change on decadal and longer time scales, and the background variability from which greenhouse warming must be detected.
One of the principal archives of climate information is the fossil record in deep sea sediments. For many years it has been known that seawater temperature plays an important role in the biogeography of many organisms living in the oceans: Tropical and polar regions foster different species. Using the fossilized remains of these organisms in deep sea sediment cores, marine geologists can reconstruct past changes in sea surface temperature and produce records that document past variations in sea surface conditions caused by changes in the earth-sun orbital geometry and by abrupt changes in ocean circulation. The major fossil-producing organisms for this type of research have been the foraminifera, single-celled animals with planktonic (floating) and benthonic (bottom-dwelling) varieties. These organisms produce an easily identified sand-grain-sized structure made of calcium carbonate (called a test) that is unique for each species and is well preserved in sediments found in nearly all of the major ocean basins.
Early 1980s studies of the geographic occurrence of the various species of foraminifera suggested that temperature was a primary control on their distribution. The observations were based on plankton tows of the upper water column and the distribution of fossilized remains in surface sediments of the seafloor below. The species could easily be grouped into tropical, subtropical, and polar assemblages (see figure at right), and the parallels with the sea surface temperature distribution were obvious. By mapping the distributions of these species, estimates of past sea surface temperatures were made and paleoceanography was born.
The accuracy of these reconstructions, however, is difficult to assess because biological systems are affected by many more environmental variables than temperature alone and because many of the environmental factors co-vary. For instance, salinity and temperature are highly correlated in the oceans because warmer waters are more likely to have experienced the evaporation that causes greater salinity. Without culturing these organisms in the laboratory (a difficult procedure that has been successful for only a few species), it is very hard to differentiate the effects of salinity changes from temperature changes. We are left with finding alternative ways to document their usefulness as temperature indicators. Using modern observational systems, including sediment traps and satellites, we are evaluating the reliability of foraminifera for temperature estimates under realistic conditions in today’s oceans.
Sediment traps are suspended on moorings to sample debris settling through the water column, debris that includes the fossil remains of planktonic foraminifera. Since sediment traps can collect time series samples with preprogrammed temporal resolutions of several weeks to several months, foraminifers, with several-week life spans, are ideally suited for collection using these moored instruments. Indeed, sediment trap samples often contain foraminiferal abundances implying a “rainfall” of several hundred to several thousand individual tests per square meter per day. Joint Global Ocean Flux (JGOFS) program studies of the North Atlantic spring plankton bloom, upwelling in the equatorial Pacific Ocean, the monsoon system in the Arabian Sea of the Indian Ocean, and the processes of the southern ocean around Antarctica provide direct observations of the sensitivity of foraminifers to sea surface temperature (and other environmental conditions). They allow us to “ground truth” foraminiferal abundance variations for paleoclimate reconstructions.
During the last decade we merged satellite observations of sea surface temperature with a global data set of sediment traps deployed by the PARFLUX research group at WHOI led by Susumu Honjo. PARFLUX sediment trap systems have been deployed in all of the major oceans basins (chart at right) and have sampled the complete range of temperature observed in the oceans today. Satellite observations of sea surface temperature, which are calculated from infrared radiation emitted from the sea surface, provide “real-time” information on changes in surface water temperatures and allow us to evaluate the changes in foraminiferal abundance in terms of temperature variations. Using the subset of samples from the North Atlantic, we have been able to directly compare foraminiferal abundances and sea surface temperatures for three of the most common and useful foraminiferal species for climate reconstructions.
The relationships between foraminiferal abundances and temperature can be dramatic. For instance, the tropical species Globigerinoides ruber (named for the pink color often found in its test) is not found in sediment trap samples when surface temperatures fall below 16°C. When the surface water warms to 23°C, the species abundances (expressed as the percentage of the organism in the sample) increase to a maximum of about 80 percent of the assemblage. In the PARFLUX North Atlantic data set, the surface water temperatures never exceeded 24°C, so we are not sure of this species’ temperature relationship in warmer waters. For paleoceano-graphic usefulness, finding this species in a sample from a sediment core can immediately determine that surface waters were warmer than 16°C; finding it in very high abundances implies that the surface waters were much warmer. Using its abundance variations in the past, we can place constraints on the surface water temperatures above the sediment core at the time the sediment was deposited. When the sediment deposits are placed within a chronology using radiocarbon dating, we can reconstruct the past variations in temperature, observe their relationships to changes in ocean and atmospheric chemistry, and determine the rate and range of natural variations in the climate system.
In the modern ocean there are more than 30 species of planktonic foraminifera, each with its own tolerance range of temperature and other environmental variables. By using the species together it is possible to constrain temperatures over the full range of conditions observed in the oceans today. For colder water species the relationships with temperature are just as striking and also have proven extremely useful. The highest abundance of Globigerina bulloides, for instance, is generally found in the mid range of surface water temperatures, from about 10 to 25°C, with highest abundances at about 17°C. This species is also found in very high abundance in regions with very high productivity caused by coastal upwelling, which complicates its relationship to temperature. For instance, G. bulloides is in high abundance in Arabian Sea waters as warm as 24°C.
The polar bear of planktonic foraminifera, Neogloboquadrina pachyderma, is found only in polar regions and comes in two forms, differentiated by its coiling direction. The left-coiling variety, which runs counterclockwise when viewed on its aperture, is found in greatest abundance in the coldest waters of the North Atlantic and is nearly absent from regions where surface waters are warmer than 8°C. The right-coiling variety is almost never present in the colder waters, but dominates the N. pachyderma population in warmer waters. Thus this coiling direction and abundance change at 8°C makes the species especially useful for tracing the past position of the 8°C isotherm. The location of this isotherm is closely related to the extent of deep water convection occurring in the North Atlantic.
Past variations in the abundance of N. pachyderma have documentedthat ocean circulation is closely linked to the rapid changes in climate observed throughout much of the last glacial interval on earth and that many of these rapid oscillations in climate occur on time scales as short as several decades. Oscillations in N. pachyderma abundance document large geographic swings in the position of the sea surface temperature fronts in the North Atlantic Ocean, swings that are apparently synchronous with large, rapid changes in air temperature (fluctuations of more than 12°C in several decades) as recorded by changes in the chemistry of ice in cores extracted from the Greenland ice sheet. These fluctuations in N. pachyderma abundance document the incursions of warm salty waters into the high latitude regions where deep water production occurs. Cooling of this salty water increases its density, causing it to sink and form deep water that flows southward at depth. This process releases heat from the surface water, causing the North Atlantic region to warm by nearly 5°C.
The extent to which we understand the link between climate changeand ocean circulation depends to a great extent on these geological records because measurements of atmospheric conditions and oceanic convection are reliable for only about the last 50 years. Though there are clearly identifiable links emerging from the atmospheric and oceanic records for this recent period, the magnitude of the changes are small compared to the large amplitude oscillations seen in the geological record. Likewise, future monitoring of changes in ocean convection and climate may also be small and will take place on time scales of 10 years or longer, thus making it difficult to identify the causes of the changes. Adding the larger, more dramatic changes seen in the geological record to the history of ocean circulation will help to constrain the factors that cause alterations in ocean circulation and increase our chances for improved prediction of future climate. But our geological reconstructions will only be a good as our understanding of the ecological factors governing today’s biota.
This research was supported by the National Science Foundation and the Office of Naval Research. In addition, the authors are indebted to the government of Iceland and the Marine Research Institute in Reykjavik for their help in maintaining several sediment trap mooring stations near Iceland. Particular thanks goes to Jón Ólafsson for his continued support and collaboration.
Bill Curry and Rindy Ostermann first met in February of 1980 on the Caribbean Island of Roatan. Bill was joining the crew of Sea Education Association’s sailing vessel Westward for a two week stint as visiting scientist. Rindy had been on board for four months as a staff scientist and notes that Bill’s midwinter Cape Cod complexion was quite striking next to the tans of the seasoned crew aboard Westward. Bill hoisted himself over the rail and headed for Rindy at the bow where he delivered a hug and a kiss from mutual friends in Woods Hole. Thus began a working relationship between the two that continues to this day. Bill is currently in the middle of a four-year term as Geology & Geophysics Department Chair. Rindy runs Bill’s lab and the Micropaleontology Mass Spectrometer for a group of five scientists. She calculates that she has spent more than five years of her life at sea collecting the samples discussed in this article.
Slideshow
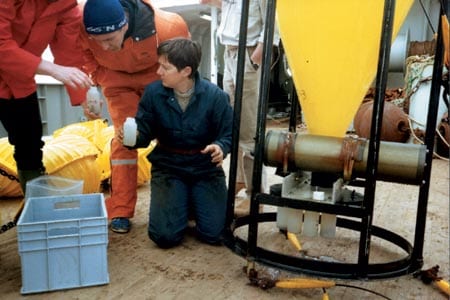
Slideshow
- Research Associate Dorinda Ostermann removes sample cups from a sediment trap recovered aboard the Icelandic Research Vessel Bjarni S?mundsson.
- Distribution maps and scanning electron microscope images for three foraminifers. The height of each bar indicates the relative abundance of the species as a percent of the total foraminifer assemblege. N. pachyderma (left coiling) on the top prefers water colder than 8 degrees centigrade. G. bulloides in the middle prefers waters that are influenced by the Gulf Stream. G. ruber at the bottom figure prefers warm central North Atlantic water.
- All biogenic particles arriving on the ocean floor carry a memory imprint of their growth environment. Fossil shells of foraminifera contain many clues to the past oceanic environment, including temperature and salinity information. The chart above shows the range of sea surface temperatures sampled by WHOI time-series sediment traps around the world from less than 2°C to greater than 32°C.The world map below indicates the locations of these sediment traps.
- Distribution of G. ruber (percent of total foraminifer population) based upon temperature of surface water obtained by Satellite data. The open circles are from the JGOFS 47 N sediment trap; closed circles are from the JGOFS 34 N sediment.
- Distribution of G. bulloides (percent of total foraminifer population) based upon temperature of surface water obtained by satellite data. The open circles are from the JGOFS 47°N sediment trap; closed circles are from the JGOFS 34°N sediment.
- The top panel shows distribution of Neogloboquadrina pachyderma (left coiling) as a percent of total foraminifer population based on surface water temperature obtained from satellite data. Abundances of this species increase in water below 8°C. The lower panel shows an abrupt change in N. pachyderma coiling direction from left to right when the surface water warms above 8°C.
- Air temperature over Greenland (based on ice core isotopic measurements) and sea surface variability in the North Atlantic (based on left-coiling N. pachyderma percentages). The covariation of the records implies that variations in the circulation of the North Atlantic are strongly linked to temperatures in the region.
Related Articles
- Paddling an angry, ancient ocean
- Reconstructing the Bering Sea’s stormy past
- Coral time machines
- Secrets in the dust
- How historic hurricanes can help predict storm intensity
- Unearthing Long-Gone Hurricanes
- Will Oxygen in the Ocean Continue to Decline?
- Blue Holes and Hurricanes
- More Floods & Higher Sea Levels