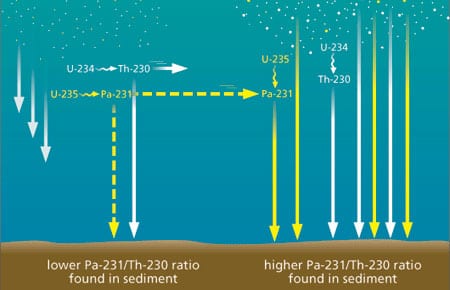
Geochemical Archives Encoded in Deep-Sea Sediments Offer Clues for Reconstructing the Ocean’s Role in Past Climatic Changes
Geochemical Archives Encoded in Deep-Sea Sediments Offer Clues for Reconstructing the Ocean’s Role in Past Climatic Changes
Paleoceanographers are trying to understand the causes and consequences of global climate changes that have occurred in the geological past. One impetus for gaining a better understanding of the factors that have affected global climate in the past is the need to improve our predictive capabilities for future climate changes, possibly induced by the rise of anthropogenic carbon dioxide (CO2) in the atmosphere.
December 1997 — Paleoceanographers are trying to understand the causes and consequences of global climate changes that have occurred in the geological past. One impetus for gaining a better understanding of the factors that have affected global climate in the past is the need to improve our predictive capabilities for future climate changes, possibly induced by the rise of anthropogenic carbon dioxide (CO2) in the atmosphere.
The relatively recent geological past (the last 1.6 million years), known as the Quaternary period, is characterized by large climatic swings from ice age to warmer interglacial periods similar to the present one. During the ice ages, large ice sheets accumulated on the northern continents, and sea level dropped by as much as 120 meters. Analysis of air bubbles trapped in the Antarctic and Greenland ice caps documents significantly lower atmospheric CO2 levels during the cold glacial periods compared to the warm interglacials (Fig. 1). Since carbon dioxide is a well known “greenhouse” gas, whose presence in the atmosphere traps heat near the earth’s surface, its lower concentration in the glacial atmosphere could have contributed to the cold climate of the ice ages.
Establishing the exact role that atmospheric CO2 played in past natural climatic oscillations, however, is not a simple matter. Changes in atmospheric CO2 may have been more a response to climate change than a forcing mechanism. On the other hand, while we know that the pace of Quaternary glaciations was primarily driven by variations in Earth’s distance from the sun and in the angle of Earth’s axis of rotation, the resulting changes in incoming solar radiation to the planet’s surface are too small to account for the large climate variability observed. This implies that the effect of these orbital parameters must have been amplified by some internal feedback mechanisms within the earth’s environment, and we suspect that atmospheric CO2 may be a major factor. In view of its obvious connection to present societal concerns, this particular problem has elicited a lot of attention in the paleoceanographic community.
In the modern ocean, factors affecting atmospheric CO2, such asexport flux of organic carbon and carbonate to the deep sea, dissolution of calcium carbonate shells in the deep sea, and deep water circulation, can be measured directly. A variety of incubation techniques are used to measure production of organic matter in surface waters, and broad views of surface water production at a given time can now be obtained from satellite imagery (Fig. 2). As several articles in this issue attest, sediment traps are deployed to estimate the export and recycling of organic matter and calcium carbonate from surface waters to the deep sea, and thermohaline circulation is becoming increasingly well constrained, both in terms of flow rates and pathways. For past oceans, however, these variables cannot be measured directly but must be inferred from proxy analysis (a marker in the sediments from which the variables can be inferred indirectly). A fraction of the biogenic particles produced in surface water survives degradation or dissolution in the deep sea and gradually accumulates on the seafloor. As many oceanic processes leave a chemical imprint in this material, a very complex but rather comprehensive chemical archive, which can be dated and deciphered, is continuously buried in deep-sea sediments.
It is probably safe to say that every element of the periodic table, every isotope, and an assortment of specific organic molecules that survive sediment burial have some potential for providing information on how past oceans operated. It is for us to discover the processes that regulate their distribution in the sediment, how well the chemical signals are preserved during burial, and whether they can then be used to infer past changes in the processes that generated them. Often, a specific element or isotope is affected not only by one but by several oceanic processes. Different elements can also be affected by the same processes, but with a somewhat different response.
Decoding this chemical message is rather like solving a jigsaw puzzle in which each piece is a puzzle in itself. You first have to build the pieces of the overarching puzzle one at a time—that is, you try to develop proxies for oceanic processes that you think may play an important role in climate control. As you keep building new pieces, you make preliminary tests to see how they come together. Oftentimes, you find that some pieces don’t quite fit and need to be adjusted. Sometimes several pieces will fit nicely together until an additional piece discloses that this was just an illusion. On good days, the added information allows you to rearrange the pieces into a more convincing pattern. On bad days, the whole thing falls apart and you are back to square one. Actually, you never go back to square one, even on very bad days, as a lot is learned from failures as well. This process, which is typical for many scientific endeavors, sounds rather daunting, at least in the initial stages, but as it gains momentum (and we have now reached this exciting part in our game), the overall picture becomes gradually clearer, and there is an inherent synergism in the process so that the emerging picture itself is giving you hints on how to build important missing pieces or rearrange those that don’t quite fit.
There is a good illustration of this process in the ongoing development of paleoceanographic tools that use natural radioisotopes formed in seawater from the decay of dissolved uranium. In view of the recognized importance of the biological pump in reducing atmospheric CO2, past change in ocean productivity is an obvious part of the “puzzle” for which a “piece” needs to be “built.” Different approaches are being explored to reconstruct that important characteristic of past oceans. Ideally, one would want a synoptic (snapshot) view of past ocean productivity, similar to what satellite data offers for the modern ocean. In one of these approaches, paleoceanographers are attempting to make use of the contrasting behavior of the two natural radionuclides thorium 230 and protactinium 231.
Weathering removes uranium from crustal rocks and rivers transport it to the ocean, which has a uniform uranium concentration everywhere. Two primary uranium (U) isotopes, U-238 and U-235, initiate different decay series and produce daughters with distinct properties (Fig. 3). Among these daughters, thorium 230 (Th-230) and protactinium 231 (Pa-231) are particularly useful for late Quaternary paleoceanography. Unlike their parent uranium isotopes, Th-230 and Pa-231 are very insoluble in seawater. They are rapidly adsorbed onto settling particles and removed to the underlying sediments through a process called “scavenging.” Such particle-reactive isotopes can be removed directly to the underlying sediment, or they can be transported laterally with seawater to be removed in regions of higher particle flux where the rate of scavenging is higher (Fig. 4). Partitioning between these two removal pathways depends on the residence time of the insoluble element in the water column. Of the two, Th-230 is the most particle-reactive. It resides in the water column for a very short period before removal, so there is very little time available for its lateral transport. Th-230 is, then, primarily removed to the sediment underlying the region where it is produced. In contrast, Pa-231 is less particle reactive and resides in the water column long enough for lateral transport over substantial distances before removal.
As a result, a significant fraction of the Pa-231 produced in low productivity, low export flux regions is removed to the sediment underlying more productive regions with high export flux (Fig. 4). Sediments underlying productive regions should thus have a high Pa-231/Th-230 ratio, while those underlying low productivity regions should have a low ratio. The validity of this model was checked by analyzing surface sediments and comparing the results to productivity maps of the modern ocean. We first looked at the Pacific Ocean, where the distribution of Pa-231/Th-230 in surface sediments compares very well with satellite imagery of ocean color and productivity (Fig. 5). Next we reconstructed export production during the last glacial period in the southern ocean (the seas around Antarctica). Comparing the distribution of Pa-231/Th-230 in modern and glacial sediments (Fig. 6) suggests that while there was relatively little change in the total productivity of the southern ocean, there was a clear northward migration of the belt of high values (and presumably high production), which is most apparent in the Atlantic sector. However, while the distribution of Pa-231/Th-230 in modern sediments again fits expectations reasonably well, the conspicuous maxima imprinted in the sediment are not as pronounced in satellite imagery. We now know that Pa-231/Th-230 in settling particles can also be significantly affected by the abundance of biogenic opal, which has a high affinity for protactinium. This piece of the “puzzle” will thus have to be adjusted accordingly. The reason this problem does not occur in the equatorial Pacific is that opal content is comparatively low in this region.
A more substantial but constructive readjustment resulted from measurement of the Pa-231/Th-230 ratio in Atlantic sediment. Just as in the Pacific, export production is high in the eastern equatorial upwelling region (Fig. 2).
We expected to find very high Pa-231/Th-230 in this region and much lower values in the central open ocean. Instead, however, low values were invariably found throughout the Atlantic, with only a few marginally higher values off West Africa (Fig. 7). This finding, surprising at first, brought to the fore the importance of an additional variable that had been largely neglected in previous work. The Pa-231/Th-230 ratio depends not only on the particle flux and scavenging intensity, but also on the rate of Pa-231 transport by currents moving from low flux to high flux regions (Fig. 4). A water mass called the North Atlantic Deep Water is produced by cooling in the North Atlantic, where it sinks before flowing southward to mix with the circumpolar deep water around Antarctica. As a result of this water entrainment, much of the Pa-231 produced in North Atlantic Deep Water as it transits the Atlantic is flushed into the southern ocean, and very little can accumulate in the Atlantic’s high productivity regions.
While it complicates the interpretation of sedimentary Pa-231/Th-230 in terms of export production, this finding also provides a means of constraining past changes in the rate of thermohaline circulation, another important piece of the atmospheric CO2 “puzzle.”
This approach was actually used to establish that thermohaline circulation was as vigorous during the last glacial period as it is today. The Pa-231/Th-230 ratio is still useful to constrain export production, but exchange of Pa-231 between ocean basins must be taken into account. Other paleoproductivity proxies, based on different principles and biased by oceanic processes other than circulation, are also being developed. By combining these different approaches, we better document and quantify glacial productivity. In turn, better glacial productivity estimates will allow us to better constrain thermohaline circulation rates from the distribution of Pa-231/Th-230.
Such iterative improvements of complementary proxies for related oceanic processes gradually lead us to an increasingly refined understanding of the mode of operation of past oceans and its influence on climate. Moreover, the paleoceanographic perspective that is being generated also provides important new insights into the working of the modern ocean and improves our forecasting ability for the future.
Funding for research described in this article was provided by the National ScienceFoundation.
Roger François started his career as a textile engineer in Zaire, printing faux-batiks on the shores of Lake Tanganyika. Looking for a more exotic occupation, he moved to Vancouver to learn the trades of oceanography and ski mountaineering, when he noticed that he was prone to being seasick and was afraid of heights. Receiving better reviews for his oceanographic accomplishments than for his telemark turns, he moved to Woods Hole to become a full-time oceanographer.
An MIT/WHOI Joint Program graduate, Mike Bacon has been on the WHOI Scientific Staff since 1977. He specializes in the use of uranium-series nuclides to study ocean processes. In his spare time he also enjoys active participation in the education of his six-year-old daughter Alexandra. Mike also enjoys studying and trading the financial markets, where he says he is still hopeful of eventually making a profit.
Slideshow
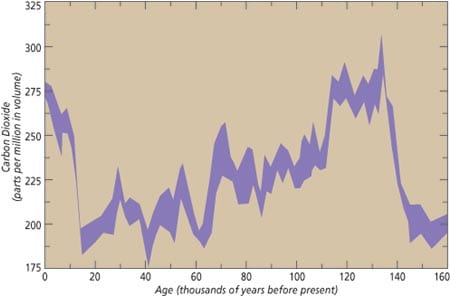
Slideshow
- Figure 1. Changes in atmospheric carbon dioxide during the last 150,000 years recorded by the carbon dioxide content of air bubbles trapped in ice that accumulated on Antarctica. (Barnola, J.-M., D. Raynaud, Y. S. Korotkevitch, and C. Lorius (1987) Vostok ice core provides 160,000-year record of atmospheric CO2. Nature 329, 408-414.)
- Figure 2. Ocean color measured by satellite. In the tropical open ocean, productivity is comparatively low due to permanent thermal stratification of surface waters that limits the supply of nutrients from deeper waters. The resulting flux of biogenic material to the deep sea is also low. In contrast, in the more productive regions of the ocean, abundant phytoplankton sustain much larger sinking fluxes of biogenic material. These regions are most prominent at the eastern margins of the oceans and at the equator, where divergence of wind-driven surface currents brings nutrient-rich water from intermediate depths to the surface. Seasonally high productivity can also be found at higher latitudes due to the seasonal breakdown of upper water stratification in winter and to deep convective mixing.
- Figure 3. Uranium decay series: U-238 initiates a decay series that produces thorium 230, while U-235 produces protactinium 231.
- Figure 4. Boundary scavenging. Thorium 230 is rapidly scavenged, that is, adsorbed onto settling particles and removed to the underlying sediments, shortly after its formation from the decay of U-234. Because of its short residence time in the water column, very little thorium 230 can be transported laterally before removal from seawater. Protactinium 231 is less rapidly scavenged from the water column and is laterally transported towards regions of higher productivity where particle settling flux and scavenging rates are higher. As a result, the ratio of protactinium 231 to thorium 230 in sediments underlying more productive regions is higher than in sediments underlying less productive regions.
- Figure 5: The protactinium 231 to thorium 230 ratio in surface sediments in the eastern equatorial Pacific. Compare the color pattern with that of area A in Figure 2.
- Figure 6: The protactinium 231 to thorium 230 ratio in recent sediments deposited in the Atlantic and Indian Ocean sectors of the southern ocean around Antarctica (compare the color pattern to area B in Fig. 2) and in sediments deposited during the last glacial period.
- Figure 7: The protactinium 231 to thorium 230 ratio in Atlantic Ocean surface sediments
Related Articles
- Paddling an angry, ancient ocean
- Reconstructing the Bering Sea’s stormy past
- Coral time machines
- Secrets in the dust
- How historic hurricanes can help predict storm intensity
- Unearthing Long-Gone Hurricanes
- Will Oxygen in the Ocean Continue to Decline?
- Blue Holes and Hurricanes
- More Floods & Higher Sea Levels