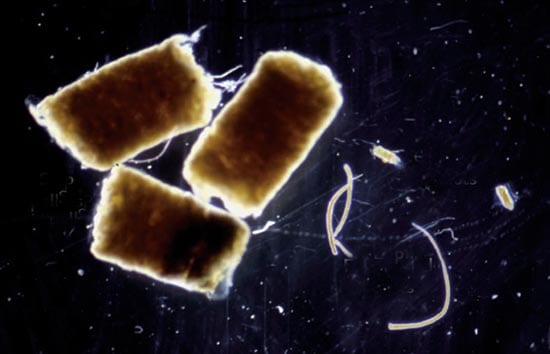
Will Ocean Iron Fertilization Work?
Getting carbon into the ocean is one thing. Keeping it there is another.
In this age of satellites, it’s fairly easy to answer the basic question of whether adding iron to the ocean can stimulate a plankton bloom. When storms over land blow iron-rich dust into the sea, satellite images show marbled swaths of green phytoplankton spinning across waters previously blue and barren. Satellites also show plankton blooms near the Galápagos and other islands where iron-rich deep waters naturally well up to surface. Even blooms spurred by experimental additions of iron to the ocean can be detected by satellite, and shipboard scientists conducting the experiments reported an almost instantaneous change in the color and even the smell of the water.
Twelve experiments so far have not looked so closely at the trickier questions of how much carbon dioxide taken up by a bloom is drawn out of the air and transferred into the deep sea, and how long it remains sequestered there. As yet, scientists have turned up only partial answers.
Philip Boyd of the New Zealand National Institute for Water and Atmospheric Research summarized the 12 experiments at an ocean iron fertilization conference convened at Woods Hole Oceanographic Institution (WHOI) in September 2007 and in an article in Science magazine earlier last year. Four took place in the northwest Pacific, two were in the equatorial Pacific, and six were in the Southern Ocean. All 12 reported up to 15-fold increases in the chlorophyll content of surface waters. (Chlorophyll is the sunlight-capturing molecule in photosynthesis and is often measured in lieu of actual plankton counts.)
Only a tiny fraction of the carbon drawn down by blooms sinks from the surface into deeper waters, where it is sequestered from the atmosphere. Estimates of the tonnage of carbon sequestered (measured at 200 meters depth) per ton of iron added hover around 200 to 1, a far cry from early experiments in laboratory beakers that yielded estimates around 100,000 to 1, Boyd said.
But those may be underestimates. Although scientists have spent up to several weeks monitoring blooms after iron addition, ship schedules and budgets have usually prevented them from monitoring long enough, or deep enough, to obtain good measurements of “export efficiency”—the proportion of carbon that sinks from the surface into deeper waters.
The 2002 United States-funded SOFeX experiment did show that more carbon was exported into deeper waters below the fertilized ocean patch, WHOI marine biochemist Ken Buesseler and colleagues reported. And unpublished results from the 2004 European EIFeX experiment showed levels of carbon sequestration that were far higher and far deeper (all the way to the seafloor) than previously observed—but this occurred only in the final days of monitoring, Victor Smetacek of the Alfred Wegener Institute in Germany told participants at the WHOI conference.
The emerging picture is that iron fertilization does in principle work well enough to squirrel away carbon for at least a few decades—possibly useful in the world’s efforts to solve its carbon emissions problem. Although present yields seem low, improved methods could boost that number in two ways: by refining logistics to make blooms larger, and by increasing “export efficiency,” or the proportion of carbon that sinks from the surface into deeper waters, where it is less easily returned to the atmosphere.
Logistics and luck
Iron addition is simple in principle, but once a ship is loaded up and heading for open waters, even small experiments become a tangle of logistics. The SOFeX experiment employed three research ships, helicopter scouts, and 76 scientists to monitor the results of adding one to two tons of iron to the ocean.
The typical method involves drizzling acidified iron sulfate into the ocean as a thin slurry, to reduce the amount that immediately sinks out of the sunlit surface waters where photosynthesis happens. Adding the iron requires a 12-hour zigzagging cruise across a theoretical square of water whose boundaries shift constantly in the ocean currents. In the weeks of monitoring that follow, a ship typically spends 12 hours out of every day just mapping out the boundaries of the bloom.
Blooms are hard to track because the added iron rapidly dilutes, sinks, and reacts with seawater, becoming virtually undetectable after a few days, Boyd said. Researchers add minute amounts of an inert tracer, sulfur hexafluoride (SF6), itself a potent greenhouse gas. (One kilogram of SF6 added in an experiment is equivalent to releasing 7 tons of carbon dioxide, estimated WHOI oceanographer Jim Ledwell, but even that is insignificant compared with the amount of CO2 drawn down by a bloom.)
“It’s really our safety net,” Boyd said. “We’ve learned a great deal about how upper ocean physics can rapidly redistribute the added iron and SF6,” which would have been hard to detect without the tracer.
Depending on local currents, blooms can wind up strewn across the ocean like a ball of string or confined within swirling loops of water known as eddies. The eventual size of blooms from small iron additions can span 1,000 square kilometers or more, extend to depths of up to 100 meters, and drift hundreds of kilometers from their starting positions. Plain bad luck can get in the way, too, Boyd said. The first experiment shut down after only five days when a mass of less dense water moved over the iron-fertilized water, pushing it far below the sunlit surface and ending the iron-induced bloom prematurely.
Location, location, location
Boyd likened a successful bloom to the real estate market. “ ‘Location, location, location’ applies equally well to these iron addition experiments,” he said. “Put it in the wrong place, and you’ll be chasing your tail across the ocean.” Blooms depend crucially on location at two levels: the appropriate ocean region as well as the particular patch of water in that region chosen to receive the iron slurry.
So far, iron fertilization has been contemplated mainly for ocean waters known as high-nutrient, low-chlorophyll (HNLC) regions. These areas have high levels of other nutrients that plankton need to grow, including nitrate, phosphate, and silicic acid. Only iron is missing. HNLC waters occur in the northern and equatorial Pacific and in the Southern Ocean, so iron addition ought to work in any of them.
Logistically, equatorial waters would be easiest to work in: It’s warm and sunny all year, the seas tend to be fairly calm, and the warm waters encourage rapid growth. But there is already enough plankton growth in equatorial waters to eventually use up their nutrient supply anyway; adding iron there just creates a faster, concentrated bloom in a specific location, but the net effect on atmospheric carbon dioxide levels is arguably negligible.
Other factors make the Southern Ocean a better choice. It has a much larger area with much higher nutrient levels, which should increase the total size of blooms that could be stimulated. It has such an abundance of nutrients that they actually sink before they can be utilized—unless more iron is supplied.
Other possible locations are the low-nutrient, low-chlorophyll (LNLC) waters at middle latitudes, where both iron and nitrate are missing. Research is less far along in LNLC waters, said Anthony Michaels of the University of Southern California, but one three-week experiment in the North Atlantic showed that adding iron and phosphorus can stimulate the photosynthetic bacteria Trichodesmium. Once armed with iron, this species can convert dissolved nitrogen gas into a usable form and possibly set off blooms as large as the ones seen in HNLC waters, Michaels said. The advantage is that such blooms add their own nutrients, rather than stripping them from surface waters—one of the key criticisms of iron addition in HNLC regions. But the blooms could quickly die out once another limiting nutrient, phosphate, is exhausted.
Once an ocean region is chosen, it pays to carefully choose a location with respect to its prevailing currents. Confining a bloom within a large, slowly rotating ocean eddy makes it easier to study, but the teeming activity may soon deplete the eddy’s waters of other nutrients, particularly silicic acid, according to Boyd, and prematurely end the bloom. Linear currents that stretch fertilized water patches into winding ribbons are harder to track, but they also tend to draw in new water, replenishing nutrients and prolonging the bloom.
Engineering a better bottom line
Logistical improvements may make it possible to spur larger blooms in the future, but how might engineering improvements make those blooms more efficient at sending carbon into the deep ocean?
One route might lie in understanding bloom ecology on a microscopic level. For example, though phytoplankton are often lumped together as “marine algae,” the term actually refers to a loose assortment of plants and single-celled organisms called protists, bacteria, and archaea, each with different sizes and biochemical requirements. Much of what ensues after adding iron depends on what species were initially in the waters. Most blooms end up dominated by phytoplankton called diatoms, whose silica shells are hard to eat. When the diatoms exhaust the water’s silica reserves, the blooms wind down, Boyd said.
The interplay among nutrients, phytoplankton, and their zooplankton predators suggests ways to improve export efficiency. Adding iron in discrete pulses may allow phytoplankton to get a head start on their predators. On the other hand, long-term additions may promote sustained blooms that sequester more carbon over time, as has been seen with studies of naturally fertilized waters, according to Stéphane Blain of CNRS/Université de la Méditerranée in France. The type of iron added is another key factor: It must be in a chemical form that plankton can easily use, not one that oxidizes to an unusable form or sinks quickly before it can be used.
Another way to improve bloom efficiency might be by targeting plankton-eating organisms known as salps. These gelatinous, colony-forming animals can act like marine vacuum cleaners, ingesting huge quantities of plankton, especially diatoms. They convert their food into large, heavy, carbon-rich fecal pellets that sink much faster than the feces or dead bodies of other zooplankton. Encouraging the growth of an indigenous species already present in the oceans would be essentially “low-intensity, free-range aquaculture,” said one proponent, Brian Von Herzen, of the Climate Foundation. Even if it were possible to cultivate phytoplankton and salps selectively, the effects of such an ecosystem manipulation remain unknown. Salps have few predators and so are something of a dead end in the food chain.
Early predictions from models of HNLC regions for iron fertilization’s potential earned attention by suggesting the technique could remove around one billion tons of carbon per year from the atmosphere at a low cost. Other estimates are lower, but none considers the LNLC areas that may also be important. But realizing such a number would require major achievements: fertilizing the entire Southern Ocean and increasing the efficiency of transferring and sequestering carbon in the deep, according to Jorge Sarmiento of Princeton University. It also ignores the likely environmental problems from such a large-scale alteration of the oceans.
As scientists and commercial outfits prepare to move ahead with experiments, questions about the realistic upper limit for carbon sequestration remain open. If iron fertilization achieves only 10 percent of the one-billion-ton-per-year potential for carbon removal, that would represent 1.4 percent of the world’s current annual carbon emissions—perhaps still a large enough number to be of use in mitigating climate change. Whether such a project would also be profitable depends on improving techniques for creating blooms in the hostile Southern Ocean. At present, predictions about what will actually happen range over “about two orders of magnitude,” Boyd said. “And that’s [a difference of] six to 600 bucks, if you want to put it on a balance sheet.”
The Ocean Iron Fertilization Symposium: Some 80 natural and social scientists from several countries—along with environmental advocates, business representatives, policymakers, legal experts, economists, and journalists—gathered at Woods Hole Oceanographic Institution (WHOI) on Sept. 26-27, 2007, to discuss the pros and cons of ocean iron fertilization as a means to mediate global warming. This series of Oceanus articles summarize the wide range of issues raised at the conference, convened by WHOI scientists Ken Buesseler, Scott Doney, and Hauke Kite-Powell. They reviewed and edited these articles, with input from many conference participants. All the articles in this series will be published next week in a print edition of Oceanus (Vol. 46, No. 1). Videos and PDF versions of presentations at the conference are available at http://www.whoi.edu/conference/OceanIronFertilization. The symposium was sponsored by the Elisabeth and Henry Morss Jr. Colloquia Fund, the Cooperative Institute for Climate Research at WHOI, the WHOI Marine Policy Center, the WHOI Ocean and Climate Change Institute, the WHOI Ocean Life Institute, and Woods Hole Sea Grant.
From the Series
Slideshow
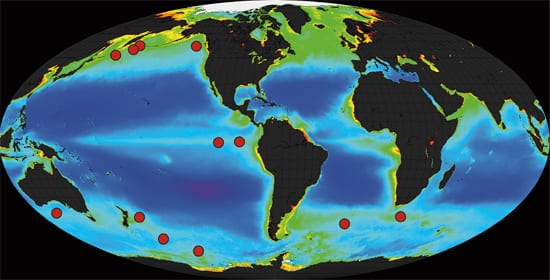
Slideshow
- TESTING THE WATERS Twelve small-scale experiments over the past decade in several ocean locations (red dots) consistently showed that intentional iron additions do result in phytoplankton blooms that help draw down carbon dioxide from the air. But the experiments have not determined how much carbon is transferred and sequestered in the deep sea, rather than quickly recycled back to the atmosphere. (From Philip Boyd, New Zealand National Institute for Water and Atmospheric Research)
- WHAT GOES IN, GOES OUT AND DOWN?A major way to remove carbon from surface waters is carbon-containing pellets excreted by organisms that eat phytoplankton. Heavy fecal pellets made by animals called salps sink faster than smaller, lighter ones made by euphausiids or copepods. (Photo by Deborah Steinberg, Virginia Institute for Marine Science)
Related Articles
Featured Researchers
See Also
- Symposium on Ocean Iron Fertilization Exploring Ocean Iron Fertilization: the scientific, economic, legal and political basis
- Fertilizing the Ocean with Iron Should we add iron to the sea to help reduce greenhouse gases in the air? (A six-part series) from Oceanus magazine
- What Are the Possible Side Effects? The uncertainties and unintended consequences of manipulating ecosystems from Oceanus magazine
- Lesson from Nature, Models, and the Past Uncertainties and unintended consequences from manipulating ecosystems from Oceanus magazine
- Dumping Iron and Trading Carbon Profits, pollution, and politics all will play roles in ocean iron fertilization from Oceanus magazine
- How Long Can the Ocean Slow Global Warming? An article by Scott Doney and Naomi Levine from Oceanus magazine
- Why Dump Iron in the Ocean? from Cafe Thorium, Ken Buesseler's Radiochemistry Lab, at WHOI
- A Journey to the Ocean's Twilight Zone A conversation with Ken Buesseler from Oceanus magazine
- Swimming in the Rain from Oceanus magazine