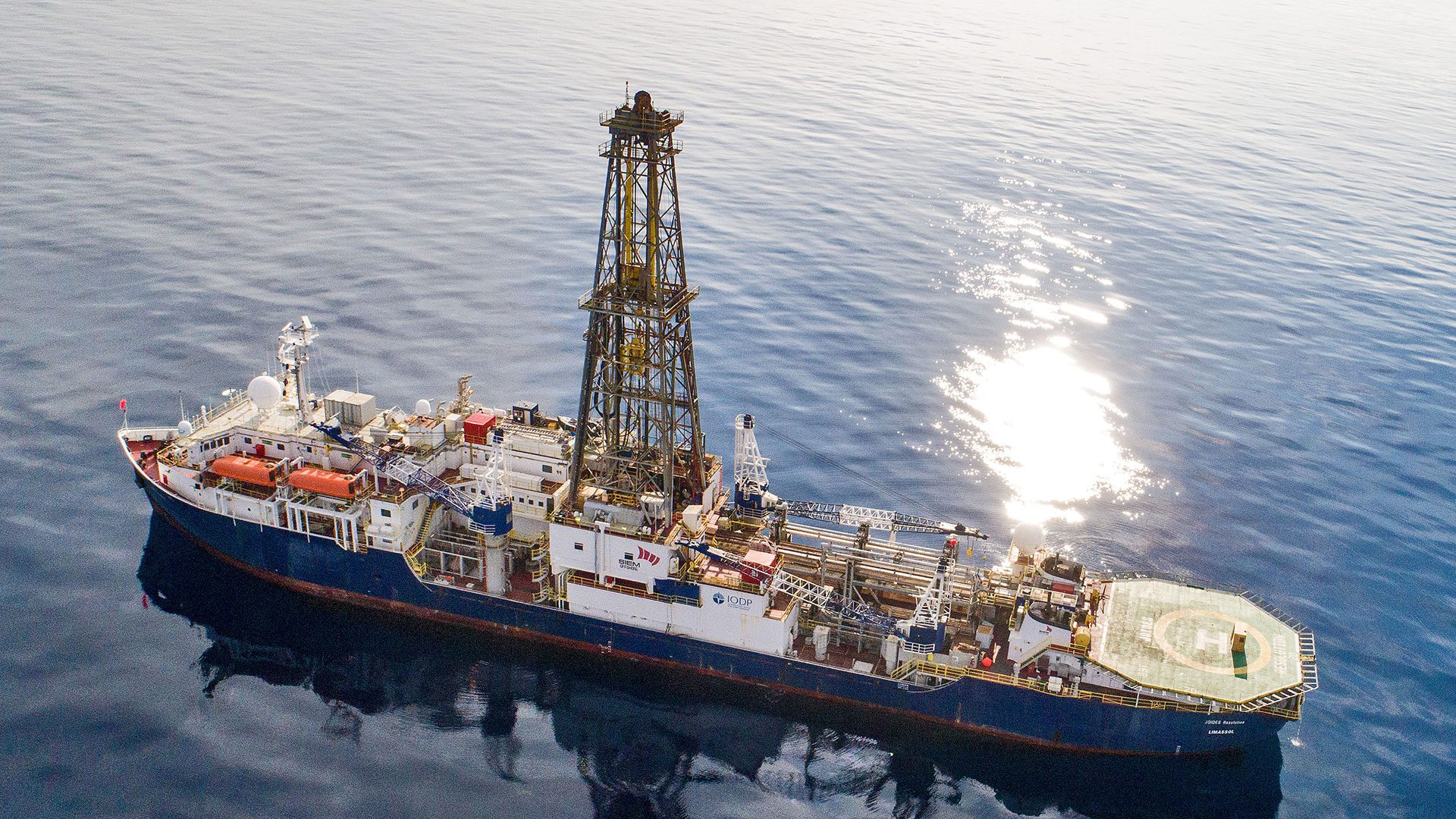
Estimated reading time: 7 minutes
From a drill ship in the middle of the Atlantic Ocean last spring, scientists drilled nearly a mile beneath the sea floor (0.78 miles or 1.3 kilometers to be exact)– the deepest hole ever drilled into mantle rock in the ocean. The record-breaking achievement was celebrated by geoscientists around the world, not least by Susan Lang and Frieder Klein, WHOI geochemists on board the JOIDES Resolution for one of the American drill ship’s final missions. The drill brought back 259 cylinders of rock and fluid samples from inside the hole, which the scientists say will provide insights into Earth’s formation– and how life may have been sparked– for generations to come.
Now that they’re back at WHOI, Lang and Klein explain how investigating this cache of inner Earth rocks is like unlocking a time capsule.
What were you most looking forward to about IODP Expedition 399?
Susan Lang: I was excited about two things. First, returning to learn more about the Atlantis Massif, which is one of the most scientifically interesting locations on Earth, in my opinion. Every time we collect new samples from there we learn something new and enlightening. Second, I have never sailed on the JOIDES Resolution before, and I was looking forward to seeing how the experts carry out ocean drilling. It is quite the operation!
Frieder Klein: For me, I was looking forward to getting access to amazing samples and meeting new friends. And not having to do certain chores like washing dishes and doing laundry! But it was difficult to leave the family at home for 2 months. Things in the lab and office at home pile up and other projects are on hold. The pressure was on: this was the final expedition to drill mantle rocks at Atlantis Massif, a large undersea mountain formed by slow seafloor spreading.
WHOI geologist Frieder Klein (right) and Barbara John (University of Wyoming) examine the cores to select samples for further analysis. (Photo by Lesley Anderson © U.S. Antarctic Program & Integrated Ocean Drilling Program)
As a co-chief scientist, why did you decide to drill at Atlantis Massif?
Lang: We decided to drill at the Atlantis Massif, in large part, because it hosts the Lost City hydrothermal field. The discovery of Lost City in 2000 was groundbreaking because it was the first example of an oceanic hydrothermal field hosted predominantly on mantle rocks. By studying the Atlantis Massif, we can examine how mantle rocks emerge from the sub-seafloor to the bottom of the ocean.
Over 20 years of research at Lost City have revealed that hydrogen, methane, and organic molecules can form there spontaneously, without any involvement from life. The chemical composition of the fluids from this field are very different from hydrothermal fields in basaltic and gabbroic rocks, which gives us incredible insights into processes that may have happened on early Earth and on other planetary bodies.
When the drill passed 500 meters below the ocean crust last spring, what was your reaction?
Klein: It was pure excitement! Although it was daunting, too, as the rock cores came on deck much faster than during any other previous drilling expedition into hard rocks, which made the workload crazy. I was on a daily 12-hour night shift from 1am to 1pm without a weekend, and the thought of continuing to drill for several more weeks at that pace was hard to fathom.
Lang: I have read stories of baseball pitchers when they are getting close to pitching a perfect game, and that everyone gets very quiet in the dugout as the game progresses. To me, it felt like that. We knew something very special was happening and it almost felt like there was a risk of jinxing it if we talked too much about it too much.
During your expedition, the drill ship reached 1267.8 meters below the seafloor, over 800 meters deeper than had been drilled before. What will this additional depth reveal about early Earth processes?
Klein: The extra 800 meters will allow us to address all kinds of open questions, such as what minerals are in the mantle, how are they distributed, and how do that change with depth? What does the chemical kitchen look like to make organic compounds from scratch?
Lang: It’s really exciting that we recovered a largely intact, very deep record of mantle rocks. Geologists have tried to piece together what the mantle looks like– compositionally and structurally– from bits and pieces that we have sampled previously, but there’s a difference between seeing the single puzzle pieces out of context and seeing a quarter of the picture assembled. Neither is a full picture, but we can build a lot of knowledge from the latter.
What is so special about serpentinite? What makes it “mantle rock”?
Klein: There are so many things that get me excited about serpentinite. Some researchers believe that a meteorite group called carbonaceous chondrites brought all of the water that we have on Earth during its formation. The main water carrier in these rocks is serpentine, a mineral that contains a lot of water. That means the water in all water-bearing things on Earth, like the ocean, lakes, rivers, clouds, and living organisms was once in serpentinite! Moreover, a byproduct of serpentinite formation (a process that we call serpentinization) is molecular hydrogen, which creates conditions where organic molecules can form and also serves as a source of energy for microorganisms that live in harsh environments. These conditions are likely similar to those on early Earth– and possibly other planetary bodies such as Enceladus and Europa, two icy moons in the outer solar system.
Mantle rocks are the source rock for basalt, which we can find on 70 percent of the planet’s surface. The mantle plays a fundamental role in plate tectonics and global geochemical cycles such as mantle convection, continental drift, the formation of continents, earthquakes, and volcanism. Understanding its architecture and composition will unlock a deeper understanding into these processes.
"The water in all water-bearing things on Earth, like the ocean, lakes, rivers, clouds, and living organisms was once in serpentinite!"
—Frieder Klein
Now that the samples are back at WHOI, what are you doing with them?
Lang: In my lab, the next steps are to analyze the samples of hydrogen and methane that we collected in more detail by determining their isotopes. That data can be used to figure out formation pathways and temperatures. In a small subset of our samples, we will also be analyzing the concentrations of small organic molecules that we believe could be created in the absence of life. These simple precursor molecules are the critical first step to lead from hydrogen and carbon dioxide to more complex organic biomolecules that catalyze the chemical reactions that make up a metabolic pathway (enzymes), carry information (DNA, RNA), and hold everything else together (cell walls).
Klein: One of the projects that I am particularly excited about will examine the chemical reactions that cause the formation of hydrogen, which plays important roles as a source of energy for microbes that inhabit ecosystems similar to what some researchers believe existed on early Earth. It also creates conditions that stabilize native metals and organic substances that some believe played a role in the emergence of life on Earth and possibly elsewhere in the solar system.
Video footage courtesy of the Integrated Ocean Drilling Program/ JOIDES Resolution Science Operator