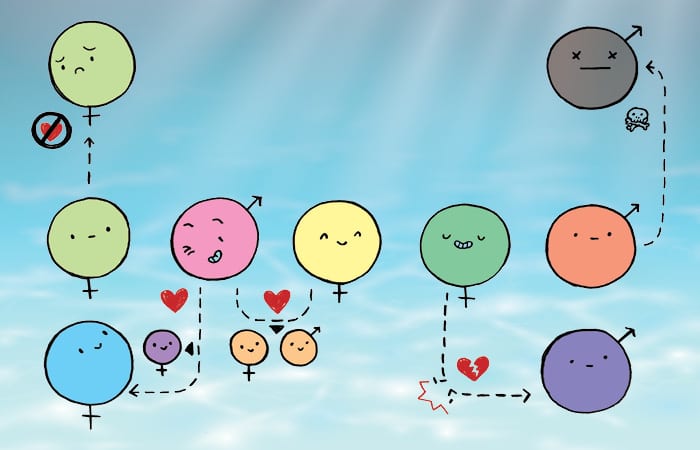
Sex, Games, and the Evolution of Gender Gaps
Population models can help us plan breeding programs for endangered species and understand the evolution of sex ratios.
Ever been unlucky in love? Well, biology might have slightly stacked the odds against you, especially if you’re a guy.
About 105 boys are born for every 100 girls, which scales up to an extra 30 million men worldwide. That makes your chances of becoming a lonely bachelor, or a very eligible bachelorette, statistically larger than you’d expect. For other species, these biological gender gaps can get even bigger. Lemmings, for example, give birth to four times as many females as males, while some ant colonies make almost all male or female eggs.
In most cases, these ratios of males to females at birth, called sex ratios, have been biologically skewed by centuries of evolution. What causes the evolution of skewed sex ratios, and whether there’ll be more males or females in the long run, are mysteries that have puzzled biologists since Darwin.
To tackle puzzles like these, scientists use demographic population models. These are mathematical equations that capture the dynamics of different groups in a population, including males and females, to project how that population will grow or shrink over time. These models are not only important tools for studying evolution, but can also be used to plan breeding programs for endangered species, set sustainable trophy-hunting quotas for big game animals, and even slow the reproduction of pests and invasive species.
Of course, population models are only as good as the assumptions we put into them. To make our models more useful, especially for understanding how gender gaps evolve, we need to figure out how evolution works and why certain sex ratios become so successful. We also need to know how small differences in sex-specific traits and behaviors can lead to much bigger population and evolutionary changes.
Let’s start by playing a game.
The mating game
Imagine you’re at a bar when you see an attractive guy or girl you’ve never met. Should you buy them a drink? Compliment their clothes? Or maybe just smile and hope they’ll notice?
According to a pickup artist, the right answer is none of the above. Pickup legend Mystery, host of the “The Pickup Artist” reality TV show, recommends infamously unconventional ways for getting attention. Think oversized top hats, black nail polish, and backhanded compliments—anything weird and unexpected that no typical person would try.
“Men are programmed to inseminate as many pretty women as possible,” Mystery’s bestselling book informs pickup hopefuls. “A good game plan expertly executed can mean the difference between replicating and having your genes unapologetically weeded out of existence.”
In other words, pickup artists aren’t trying to find their one true loves. They’re playing a game to snag as many mates as they can. And to win hearts, or at least a few phone numbers, they need what Mystery calls a good “game plan,” a strategy that’ll make wannabe seducers stand out from the crowd.
Evolution, it turns out, is a similar game, albeit one with much higher stakes. The players in the evolution game aren’t just people in a bar; they’re all the individuals in a species. Each individual’s “game plan,” or strategy for success, is a genetically encoded set of traits called a phenotype—not so much crazy clothes or gimmicky pickup lines, but innate biological characteristics (body size, number of offspring, disease resistance, etc.) that determine one’s desirability as a mate.
In many cases, a phenotype’s success depends on its frequency relative to other phenotypes in the population. Evolution under these conditions is called frequency-dependent selection. Some phenotypes become “fitter” as they become more common (positive frequency-dependent selection). For other phenotypes, rarity is the key to success (negative frequency-dependent selection). Mystery’s winning game plan—behaving very differently from normal individuals—is an example of the latter.
Win or die
A species’ ultimate goal—to survive and grow—hinges on the success of its phenotypes. In the evolution game, you win by having a phenotype that helps you survive, mate, or reproduce your genes more than the competition. Winners thrive, spreading their successful phenotypes to future generations. Losers, as Mystery would say, have their genes “unapologetically weeded out of existence.”
Winners can’t rest on their laurels for long, though. In the same way that newcomers with snazzier outfits and snappier lines could challenge old-timers like Mystery, even established phenotypes are subject to new mutations. And if an upstart mutant does better than the reigning champion, it could very well take over as champion itself.
Shifts in the champion phenotype are what we see as evolutionary change, and the traits we have today are those of the longest-running champs. These are the strategies that have triumphed against countless competitors. They are fail-safe game plans that have beaten out many past mutations.
But even so, isn’t there always the risk that someone, someday, will come up with a better game plan? As long as new mutations keep coming, the game will never end, and evolution will never stop.
Right?
Boys versus girls
We can answer this question by playing the evolution game for sex ratios. In this game, your strategy is the ratio of male to female children that you produce. As always, your goal is to spread your genes as much as possible.
Is your best bet to make boys? You have the edge if your competitors make mostly girls, since your sons will be highly in-demand mating partners (think a male exchange student at an all-girls school) who can give you lots of grandchildren. But if the other parents are pumping out sons, your boys are no longer that valuable, because girls have become the hotter commodity.
Simply put, your game plan depends on what everyone else is doing. Or, to be precise, what everyone else isn’t doing. Just as pickup artists use weird clothes or wacky conversation starters to stand out from the crowd, your best bet in the sex ratio game is not to conform. It’s to provide what no one else will—in this case, offspring of the rarer sex. In evolutionary terms, sex ratio evolution is a classic example of negative frequency-dependent selection.
As in all cases of negative frequency-dependent selection, success has a hidden downside. If your strategy gets too popular, it’ll go from new and edgy to old and overused. Son-producing parents thrive when males are rare, producing many descendants who also favor sons. Those descendants will produce more and more sons themselves, until boys are less in demand. At this point, the rarer girls gain the upper hand. But once those girls get too popular, the tables turn once again.
This popularity tug-of-war goes back and forth until things finally even out. Once there are half males and half females, the game reaches a stalemate. Male and female offspring are now in equal demand, so a male- or female-biased sex ratio won’t give you any advantages. At this point, it doesn’t pay to go against the crowd. If all the other players are using it, the 50:50 strategy is unbeatable.
Biologists call unbeatable strategies like the 50:50 sex ratio evolutionarily stable strategies, or ESSs for short. Another name for an ESS is an “evolutionary trap.” This is because once an ESS spreads throughout the population, it is completely invincible. No other strategy can do better than the ESS, so all competing mutations eventually die out.
In other words, once an ESS appears, the game is over. The population is stuck at that phenotype without any further evolution.
The ideal sex ratio
That brings us back to our age-old question. If the 50:50 sex ratio is an unbeatable evolutionary trap, why are there non-50:50 sex ratios in nature?
Well, our game so far actually made several big assumptions. For one, we assumed that couples pair up one-to-one, so that everyone mates if males and females are equally abundant. But real life isn’t so simple. For polygamous species, in which one male mates with many females or vice versa, the mating scene is less straightforward. We also assumed more mating opportunities means more reproduction; in reality, matings may end with widows or divorce rather than viable offspring.
Most importantly, we treated males and females as equally valuable to evolution. This is true only if both sexes have similar traits and lifestyles. In many species, one sex is favored over the other because of differences in how males and females grow, survive, and reproduce.
In humans, for example, baby boys historically have had more health complications than girls. Since boys were more likely to die young, fewer males would be available for mating. This gave parents with sons an advantage, allowing male-biased sex ratios to outcompete the 50:50 strategy. As a result, the ESS sex ratio for humans skews slightly toward males. Whether it’ll eventually return to 50:50 with reduced infant mortality, or if other factors will still favor males, remains to be seen.
Building better models
In models, as in life, the devil is often in the details. As different individuals travel from birth to mating to death, they experience many different factors that interact to shape the fate of the entire population. One sex may have higher mortality rates, longer maturation times, or require more resources from their parents to reach maturity. All these factors affect how many males and females, both young and old, will survive and compete to reproduce. This, in turn, determines everything from future population growth and composition to long-term evolutionary trajectories.
Tracking all the possible sex-specific differences as individuals are hatched, matched, and dispatched is no small task. That’s where my research comes in. I develop models that capture increasingly complex life histories, including sex-specific characteristics and behaviors that occur at different points in the life cycle. This allows me to simulate anything from human marriages and lion harems to variations in male and female infant mortality rates or hunting levels.
As a result, my models can be applied to many conservation and management problems, as well as to evolution. In particular, I’ve used them to figure out which sex ratios will become unbeatable ESSs in various populations. With my ESS calculations, I can show how even small life cycle differences have much bigger consequences for evolution —sometimes in ways we wouldn’t expect.
For instance, I’ve found that simply changing the timing of male or female mortality can impact sex ratio evolution. When males are more likely than females to die as infants, the ESS sex ratio is male-biased (more males are born). But if males are more than females likely to die as teens, then the ESS sex ratio becomes female-biased (more females are born). These surprising results depend on the intricacies of complicated processes like parental care and mating, which are difficult to decipher without some helpful mathematical tools.
Ultimately, models like mine can help us better understand how sex ratios affect both ecology and evolution, which ESS sex ratios will come out on top, and maybe even our own descendants’ odds of finding a mate—as determined by evolutionary factors that pickup artists could never even imagine.
This research was funded by the National Science Foundation, Woods Hole Oceanographic Institution Academic Programs, and the European Research Council.
From the Series
Related Articles
- Behind the blast
- Seeding the future
- Cold, quiet, and carbon-rich: Investigating winter wetlands
- Sonic Sharks
- A rare black seadevil anglerfish sees the light
- How will we ever count them all?
- Five marine animals that call shipwrecks home
- Deep-sea amphipod name inspired by literary masterpiece
- The case for preserving deep-sea biodiversity