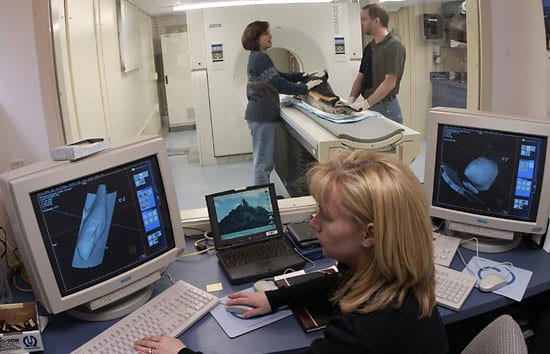
How to See What Whales Hear
Biomedical imaging reveals new insights into marine mammal ears
On summer nights, if you sit quietly at the edge of a field or watch the edges of the light pools around street lamps, you will see bats swooping through shadowy darkness in search of moths or other flying prey. They detect and catch their targets through echolocation, or biosonar, the animal equivalent—and precursor—to man-made sonars.
Bats generate signals in their nose and throat that produce echoes, which the bats monitor to determine the size, shape, speed, and direction of their prey, as well as any other objects in the area. Biosonar is also how they navigate in dark caves. Bats’ large, distinctive, convoluted, and mobile ear flaps are critical for the fine-grain acoustic analysis they do during echolocation.
Now flood that field with seawater and make it not only dark, but profoundly deep and filled with a myriad of exotic creatures and objects. That is the dim and complex world in which whales live.
Although whales and dolphins are air-breathing mammals, they spend approximately 85 percent of their time submerged. Compared to sound, light does not penetrate water well, and it is not surprising that whales and dolphins rely primarily on hearing and sound rather than sight to sense their environment and communicate. The Odontoceti—toothed dolphins and whales that hunt fish, squid, and other prey—evolved parallel abilities with bats, actively using clicks and pulsed sounds for underwater echolocation.
However, there is one very striking difference between bats and dolphins. The latter appear to have no outer ears. Dolphins and whales abandoned external ears as a concession to better underwater mobility. Still, they do have ears buried inside their heads: fascinating ears in fact, with exceptional range that operate at extraordinary depths.
With the help of a common medical tool—biomedical computerized tomography, more commonly known as CT and MRI scanning—we are beginning to get inside the heads of whales and dolphins. Using biomedical imaging techniques, we can thoroughly explore just how their ears are constructed—and see how and what they hear.
Into the inner ear
Whale hearing is difficult to study by conventional methods. Whales are large, elusive, diverse creatures, and research on most species is substantially restricted because of their endangered status. One approach to learning how whales hear is by reverse engineering, which is essentially the clockmaker’s child approach to science. We can examine stranded animals to determine not only what may have caused their deaths, but also, literally, what makes them tick.
Virtually all mammals have the same three basic ear components: an external ear flap, or pinna, is connected via an ear canal to the middle ear cavity, which has an eardrum and bony lever system for amplifying sounds, and then an inner ear, which transduces sounds into neural impulses. In marine mammals such as seals and otters, the pinnae are reduced to allow them to swim faster; in whales and dolphins, the sleekest and fastest of marine mammals, these outer ears are gone completely.
Traditionally, investigating the inner workings of whales and dolphins has been done by dissection. But to examine the parts, you are compelled to disassemble the relationship of those parts, which is fundamental to understanding their effective operation. Even worse, conventional dissection requires time, especially when the subject outweighs you by several hundred pounds. By the time you can get to many structures, they have deteriorated beyond recognition.
We knew there are robust, complex inner ears buried deep in dolphin and whale heads, but we did not know how sound gets into those inner ears.
Seeding an innovative idea
At WHOI, we took a different approach, or rather, we updated the traditional one. We still dissect, but our dissections are digital. Biomedical scanning rapidly and non-invasively reveals in great detail the internal structure of the object or animal that is imaged. We can use imaging techniques to see into most living animals. To image rare and deep-ocean materials, we do not need to remove them from their protective containers.
Above all, we see the synergy of the structures. For ill or stranded animals, we can locate and examine pathologies or traumas non-invasively—precisely what scanners were designed to do for humans.
The idea of using a CT scanner to probe inside marine mammals was a radical idea a decade ago. In 1998, with funding from the Andrew W. Mellon Independent Study Award program at WHOI and from The Seaver Institute, the first large-scale study of marine mammal auditory systems using computerized tomography was undertaken using CT and MRI scanners in area hospitals. This study demonstrated the extraordinary potential of scanning for marine mammal research, since it allowed high-resolution anatomical surveys of many individual animals in an unprecedentedly short time.
In 2000, the Office of Naval Research (ONR), and particularly Admiral Paul Gaffney, former Chief of Naval Research, furthered the effort by providing start-up funds to install a high-capacity CT scanner at WHOI that was dedicated exclusively to marine research.
How CT scanners work
CT scanners use X-rays to produce an image of density differences of internal structures. The denser an object, the less X-ray energy is transmitted to the detector, and the brighter the object in the image. For this reason, bone appears white, air looks black, and soft tissues are varying shades of gray in an X-ray.
In common single plane X-rays, such as chest films, the detector is a sheet of film that is exposed by a single pulse from the X-ray tube. Consequently, the output is a flat image in which one structure overlays another.
CT scanners employ a bank of electronic detectors that monitor the X-ray attenuations from multiple pulses and positions, as the X-ray tube moves through an arc around a patient or specimen. This complex, multi-dimensional matrix of attentuations is then deconvolved to generate images that represent the attenuations in thin cross-sections.
It is, in a very real sense, a virtual dissection, slice by slice, of all structures. The WHOI scanner allows us to image slices as thin as 0.1 millimeters and to detect attenuation differences that are several thousand-fold.
Using scanning techniques to look at whale and dolphin ears, we can study the geometry and composition of ears and other head tissues from microscale to macroscale and thereby gain insights into what and how they hear. We also see sometimes how they were damaged.
The impacts of sound
Sound is energy. The louder a sound an animal can hear, the greater the potential for damage to its ear. Some loss of hearing from day-to-day wear and tear is normal; some is excessive and avoidable, as far too many of us are well aware from exposure to loud music, power tools, or other intense sound sources.
However, just to complicate matters, not every sound is equally dangerous to all ears. Because different species have different hearing capacities, what is imperceptible to one animal may be annoying or even harmful to another. An ultrasonic dog whistle is imperceptible to humans but clearly heard by any normal dog or cat.
Even more important, the effects of sound can range from the physical, with actual damage to parts of the auditory system, to behavioral: sounds so disturbing that animals abandon normal activity, such as feeding and breeding, or even alter their migration paths.
Both physical and behavioral effects potentially have serious impacts on individuals or on entire species. Consequently, understanding hearing in marine mammals is not just a matter of curiosity, but fundamental for marine conservation and possibly even for the survival of some species.
Ships, sonars, and strandings
The ocean is a naturally noisy place. Sounds are generated by vulcanism, wind, waves, seismic events, and by animals themselves. However, all human activities in or near the water are adding to this natural suite of oceanic sound.
In recent years, mass strandings of whales—in Greece in 1995, the Bahamas in 2000, and the Canary Islands in 2002—have focused attention on the possible effects of man-made sound in the oceans. In those cases, multiple U.S. and NATO ships were engaged in exercises employing multiple and intense sonars in narrow straits.
While the presence of these ships and the exceptional sound field produced by the exercises clearly coincided with the strandings, we are not yet able to determine exactly what mechanism led to them. We examined many of the stranded animals using our scanner system and found distinctive traumas, but the damage is not strictly acoustic. Rather, it appears to be more consistent with stress than directly sound-induced.
In other cases, however, we have found damage to ears, often from aging or long-term noise exposures that clearly impaired the animals’ hearing and therefore their ability to function in the wild. At this point, we do not know precisely what noises are most harmful, either directly or indirectly, to any marine mammal species, but this is a critical area of research that we must pursue intensely and rapidly.
The inside story of dolphin ears
One of our first major discoveries answered the original mystery of the missing external ear: Without external pinnae and no obvious canal, how does sound enter dolphins’ heads and how does it get to the inner ear?
Researchers had speculated that since dolphin inner ear bones were located near their jaws, perhaps the soft tissues and bone of the jaw played a role. Unfortunately, that was hard to prove because fat tissue in the area deteriorated rapidly, and the relationships between tissues were disrupted as soon as they were cut during dissections.
CT scanning gave us the first undisturbed images of this region. In fact, it provided the critical clues: The fatty lobes near the jaw were connected to the ear and had shapes similar to bat pinnae. In effect, bats and dolphins seem to have parallel ear evolution. Dolphins have pinnae that are just as complex and large as bats, but they are internal—an advantage under water both hydrodynamically and functionally; these specialized fats have acoustic properties similar to seawater. Consequently, in terms of both shape and physics of sound in water, they are the aquatic analog of land mammal outer ears that were designed to capture and conduct air-borne sound.
The speed of sound in water
Scanning also allowed us to measure the locations of dolphin ears in situ, which explained why the ears are spread so far apart in dolphin heads. Dolphin ears are widely separated to accommodate the speed of sound in water, which is 4.5 times faster than in air.
One clue to determining the location of a sound source is the difference in arrival time between your ears. Humans have trouble locating sound sources under water, because, acoustically, our heads “shrink” nearly five-fold because of the increased speed of sound through water. As dolphins evolved, they expanded their heads and inter-ear distances to match sound speeds in water, which explains their extraordinary ability to localize sound sources three times better than humans.
Scanning also provided the first data on the inner ear of the true behemoths of the oceans. Blue and fin whale ears are massive. Their inner ear bones are approximately the size of a human brain case and are at least twice as dense. To demineralize these ear bones in order to dissect them by traditional methods would take more than two years. With scanning, we can digitally slice them to see inner ear features in less than one hour.
Anatomy reveals hearing capacity
Although all mammal ears have the same basic parts, there are important differences among species in some structures that account for differences in their hearing capacities. No two species have exactly the same hearing ability. Different animals can detect different frequency ranges and have different sensitivities at any one frequency. Most mammals hear frequencies well above the range of human hearing, termed ultrasonics. Some also hear well at very low frequencies, even at seismic sounds generated by earthquakes.
To study both normal and abnormal hearing, our laboratory has used the scanner to image all parts of the auditory system of more than 30 species of marine mammals. Each ear from an unknown hearer is compared with those from species with well-documented hearing characteristics. In particular, we construct “maps” of the stiffness and mass of ear components of animals whose frequency ranges are known and compare the stiffness and mass of newly imaged marine mammal ears to calculate their resonant frequencies. Thus, we can determine the critical commonalities for hearing in all mammals—as well as critical differences for specialized hearing like echolocation and for hearing under water instead of in air.
We also make maps this way for the few species of marine mammals for which hearing has been tested. These are our model controls, as our maps are consistent with audiograms or hearing curves of the tested animals. The new ear maps from untested species have led to the discovery that whales have some of the widest hearing ranges of any mammal and that some species are capable of hearing at seismic or hyper-ultrasonic frequencies.
We now know that some species of whales have a 12-octave hearing range, compared to eight in humans. Some whales hear well down to 16 hertz (or cycles per second), versus our lower limit of 50 hertz, while others hear as high as 200 kilohertz. The typical human high-frequency cutoff for humans is 16 kilohertz. For bats, it is 60 to 70 kilohertz.
This work is coordinated also with other WHOI laboratories doing basic research on marine mammal sounds, diving, and foraging behaviors, as well as applied research on acoustic devices to warn highly endangered species of impending ship strikes. So far, we know there is no single sound bite that is perceptible or harmful to all marine creatures, but with luck, we may be able soon to provide guidelines that will help preserve some of them.
CT scan menagerie
Peering into whale headswithout the loss of tissue and time that normal dissections causewas the initial motivation for using a CT scanner for marine mammal research, but our current scanner has had more than its share of other types of species and objects. Some of the specimens scanned here, particularly to assist the work of other researchers, have been remarkable.
Scan data obtained at the WHOI facility have proven invaluable for investigating everything from diagnosing sinus infections in live, sneezing seals to imaging shark balance organs, coral reef fish swim bladders, flippers of all forms, fractures in great whale jaws, coral reef growth patterns, pressurized ocean sediment cores, and, most exotic of all, the complex mineral substructure of deep-ocean chimneys.
Animals as small as grass shrimp have been scanned to assist modelers to determine how much sound energy large groups of similar invertebrates, called krill, reflect at different frequencies. Acoustical oceanographers use such models to determine whether reflected signals at sea actually represent deep layers of millions of krill in patches throughout the oceans.
Land creatures that also have been scanned in the last two years include tigers, hedgehogs, bats, and even an elephant and a hippopotamus (parts onlythey are just a tad too big for a whole one to fit on the table).
In 2005, the scanner is scheduled to move into a new facility on the WHOI Quisset campus. Moving the scanner is not trivial; in fact, the scanner has a good deal in common with the megalithic money on Yap. Both are giant toroids that, once in place, are daunting to shift.
The scanner move will require two engineers, a rigging crew of up to six workers, and two weeks of disassembly and reassembly time. Still, the effort will be worth it, as the new facility incorporates overhead hoists and tracks connecting the scanner room with surgical and storage facilities that will allow us to transport, scan, and understand an even wider range of marine creatures that may come our way.
Slideshow
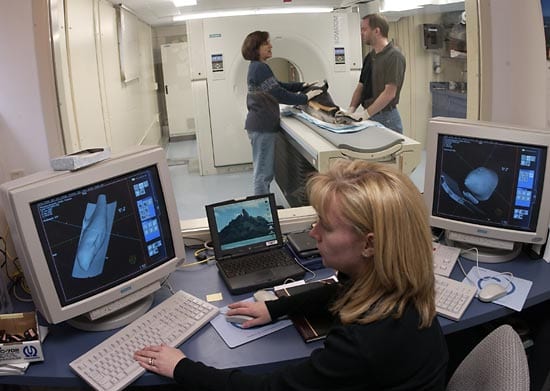
Slideshow
- READY TO SCAN—Postdoctoral Investigator Soraya Moein Bartol and Senior Research Assistant Scott Cramer position an Atlantic white-sided dolphin, which stranded and died, on the WHOI CT scanner bed before imaging, while CT technologist Julie Arruda (front) examines previously generated images. (Photo by Tom Kleindinst, WHOI.)
- INTERNAL EARS—A 3-D image generated from a CT scan highlights selected tissue groups of a bottlenose dolphin?s head. It shows the relationships of the exterior skin (blue), brain (pink), inner ear bones (red), and specialized auditory fats (orange). The fats form paired lobes inside the head along the jaw and are very similar in shape to the outer ear flaps (pinnae) of bats. (Courtesy of Darlene Ketten, WHOI)
- VISIBLE HEARING?This 3-D CT scan image of a blue whale?s inner ear (18 millimeters in diameter) shows typical mammalian inner ear structure, including a spiral cochlea and the vestibular system that controls balance. (Courtesy of Darlene Ketten, WHOI)
- A SECRET SEEN?This 3-D image shows an intact, near-term fetus discovered inside an Atlantic white-sided dolphin that stranded and died. The fetus?s flippers are folded and its ribs are lightly mineralized, but the cross-section reveals fully matured ears. (Courtesy of Darlene Ketten, WHOI)
- RARE VIEW—CT scan of an entire Kogia (pygmy sperm whale) illustrates the advantages of this instrument. It reveals the skin and outer structure (including fins), and the fully articulated skeleton in its normal configuration, from any selected perspective, without cutting into the specimen. (Courtesy of Darlene Ketten, WHOI)
- CLOSE TO HER WORK—Wearing fisherman?s cold-water gear, Darlene Ketten (left) stands in the mouth of a stranded humpback whale towed ashore on a Newfoundland beach in 1998. Working as quickly as possible, she and Jon Lien of St. John?s University, Newfoundland, remove biopsy samples and the inner ears to determine if it was injured by nearby explosions for a construction project. (Courtesy of Darlene Ketten, WHOI)
- A mandible (lower jaw bone) of a North Atlantic right whale is prepared for scanning by MIT/WHOI Joint Program graduate student Regina Campbell-Malone and CT technologist Julie Arruda.
Related Articles
- How WHOI helped win World War II
- Learning to see through cloudy waters
- Are warming Alaskan Arctic waters a new toxic algal hotspot?
- 5 essential ocean-climate technologies
- A curious robot is poised to rapidly expand reef research
- A new way of “seeing” offshore wind power cables
- New Techniques Open Window into Anatomy of Mollusks
- The Deep-See Peers into the Depths
- Re-envisioning Underwater Imaging