How Did Earth Get Its Ocean?
A student’s quest to learn the origin of our planet’s water
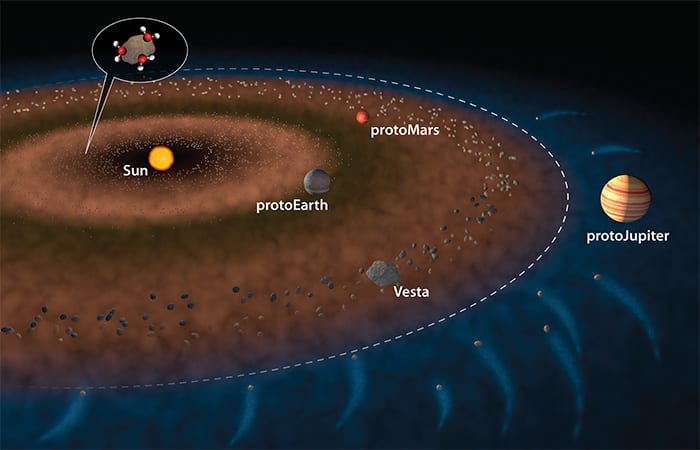
Estimated reading time: 14 minutes
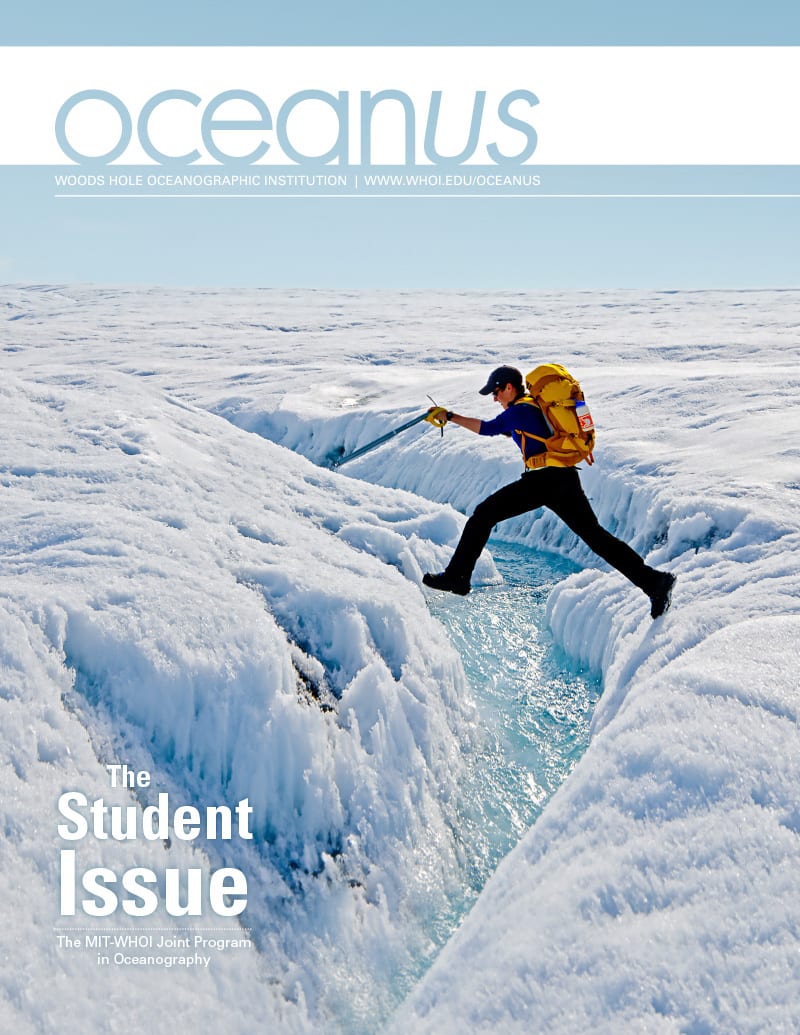
Adam Sarafian came to Woods Hole Oceanographic Institution (WHOI) as a graduate student to learn how Earth got its ocean in the first place.
“The big question is, how and when did the planet get its water?” said Horst Marschall, one of Sarafian’s Ph.D. advisors at WHOI. “All peoples have myths about where water came from. In Genesis in the Old Testament, in Norse myths, and Greek myths too—it’s an old question.”
Indeed, it’s one of the oldest questions in the solar system, and there are two possible answers, Sarafian said: “Either the Earth was formed and dry, sitting there, waiting for water, and the water came from comets or other wet bodies hitting Earth relatively late in history. Or, the water came from inside Earth—meaning Earth got its water while the Earth was still forming, and then volcanoes out-gassed steam and other water-containing compounds to the surface.”
Ironically, answers to the mystery lay not in liquid water but in solid rocks. Sarafian and colleagues pursued a painstaking path to extract evidence from rare samples of ancient meteorites that had fallen to Earth.
But Sarafian was no stranger to persistence. He overcame a learning disability that makes it hard for him to read and surmounted heights as a an All-American pole-vaulter—all before launching a scientific career that has now allowed him to hurtle across the universe and back through time to the period when Earth was still forming.
Before launching his scientific career, Adam Sarafian was a champion pole vaulter in high school and college. (Steven Sarafian)
Heights and hurdles
In the third grade, Sarafian was diagnosed with a learning disability. “I couldn’t really read a paragraph and know what the paragraph said—any paragraph,” he said. “Instead of taking electives, I took special education classes. It’s been a struggle throughout school—just trying to get myself to be able to read.”
But he got to be pretty good in athletics. “My mother owns a gymnastics school in Eatontown, New Jersey,” he said. “I grew up in the gym. I hung out there and played around when my mother was working.”
His high school’s track coach coaxed him to try pole-vaulting, and on a lark, he did.
“By my junior year, I set my goal to win the state championships,” he said. “So I trained nonstop.”
“Adam was meticulous in his preparation and attention to detail,” said his coach, Mark DeSomma. “He knew all the rules and regulations. At our conference championship meet, he informed the meet director that the pole vault pit was not regulation. The meet director told him, ‘Son, you can choose to jump or not jump, but that is our pit and it is not going anywhere.’ ”
Sarafian proceeded to break the state record of 16 feet 6 inches, which had stood for 25 years. Then he went on to jump 17 feet 4½ inches at the conference championship meet, vaulting over the record by an astonishing 10½ inches.
That earned him a pole-vaulting scholarship to the University of Georgia, where he became an NCAA All-American. By his senior year, however, injuries mounted.
“I broke my hand, when I broke a pole. And I said, ‘It’s my last year, I might as well just keep going. We’ll tape the hand up, it’ll be fine.’ Then late in the season, my feet started hurting really bad.” Sarafian completed his last two months of his NCAA track-and-field career with fractures in both feet.
Track and fieldwork
At the regional championship meet, Sarafian could barely walk. “After each jump I couldn’t even walk off the mat. I’d crawl off. I thought, ‘Well, I won’t make the nationals, but it’s been a good ride.’ ”
He was somewhat relieved for another reason. In college, he had started to develop another passion: geology. Graduating with a geology degree required two months of fieldwork, which began about the same time as the national championship meet. “Then the official comes over and says, ‘You made it to nationals!’ ”
In the preliminaries at the nationals, his feet were so swollen he wore a bigger shoe size.
His feet hurt so much, he could bear to take only short runs up to the bar. “I was like, ‘All right, last jump, this is it!’ I did it, and said, “At least I made it to the nationals preliminaries.’ Then the official walks over and says, ‘You made it to the finals!’ and I’m like, ‘Noooooo! I have to go do fieldwork.’ ”
At the last minute, however, he was cut from the competition. “It was bittersweet getting cut, but I knew I was not at all at my top, and my body was breaking. I watched the finals and cheered on all my friends. Two days later, I was in Alaska wearing hiking boots, climbing mountains, doing geology fieldwork.”
“The pole-vaulting event,” DeSomma said, “is repetitive, measured, with many frustrating days, narrowly missed attempts, and failure after failure, until those amazing moments of success. Adam’s personality was one of relentless pursuit of excellence.”
Sarafian channeled that energy into his post-vaulting scientific career.
Adam Sarafian prepares samples of meteorites to remove all contamination from the sample surface in the clean room in WHOI scientist Sune Nielsen’s NIRVANA (Non-traditional Isotope Research on Various Advanced Novel Applications) lab. (Jayne Doucette, WHOI)
In the beginning
The question about the origin of Earth’s water first arose in Sarafian’s undergraduate classes and continued as he pursued a master’s degree at Georgia. “The answer was always ‘we don’t know!’ ” he said.
In the nascent days of our solar system some 4.6 billion years ago, Earth and other protoplanets were still taking shape, Sarafian explained. Within a certain distance from the sun, it was too hot for water to remain stable, and any vapor would have been blown out by solar winds. Beyond a distance far enough from the sun, called the “snow line,” water could exist in the form of ice. Encircling the inner edge of the snow line was a belt of asteroids that included a large one called Vesta. “It’s almost like a planet that didn’t fully form,” he said.
About 15 years ago, “scientists began thinking that maybe Earth’s water came from carbonaceous chondrites,” Sarafian said. These are a type of meteorite that contains lots of water. The hypothesis was that the orbit of the vast protoJupiter began migrating closer toward the sun. “Jupiter said, ‘Get out of my way’ and all these water-rich carbonaceous chondrites outside the snow line got flung toward the sun, and all the inner planets, Mercury, Venus, Earth, and Mars. They would slam into Vesta or even the Earth and get incorporated into rocks in the inner planets within the first 20 million years of solar system formation.”
So scientists began comparing Earth’s water with the water in carbonaceous chondrites. The key is hydrogen, the most abundant element in the universe. Hydrogen has two isotopes—normal hydrogen, with a mass of one, and deuterium or “heavy hydrogen,” with a mass of two. The ratio of these isotopes differs in different parts of the solar system. The sun is made mostly of normal hydrogen. But comets, made mostly of rock and ice, formed much farther from the sun and are richer in deuterium. The hydrogen in Earth’s water is somewhere in between the sun and comets.
Measurements of hydrogen isotopes in carbonaceous chondrites matched very well with Earth’s water. That gave credence to the idea that Earth’s water came from chondrites. But when did this occur? The problem was that chondrites could have brought in water early, slamming into the growing planet, or late, pelting Earth after it formed. To know, scientists needed to find water in rocks that formed very early on, in the same region and time as Earth.
A promising source was a type of rock called eucrites. These are pieces of the asteroid Vesta that have fallen to Earth in the form of meteorites.
“Vesta completely froze and locked up about 14 million years after the start of the solar system, so it got all its water before then,” Sarafian said. “At the time, the Earth was one-quarter to one-half its size and still growing.”
To pursue his quest, Sarafian needed to jump two obstacles: He needed to get rare samples of eucrites, and he needed to find a way to measure the water in them.
WHOI scientists are known for the fact that many of them address some of the most immediate and pressing questions of our time. MIT-WHOI graduate student Adam Sarafian, however, is asking a question so old that it appears in many creation myths: How did Earth get its water? Here, Sarafian holds a sample of a meteorite loaded into a disc and ready for analysis in the Northeast National Ion Microprobe Facility at WHOI. His work is strengthening the link between Earth's water and minerals locked in rocky bodies such as the asteroid Vesta.(Photo by Jayne Doucette, Woods Hole Oceanographic Institution)
An appetite for apatites
To get eucrites, Sarafian petitioned institutions that collect meteorite samples, such as NASA, the Smithsonian Institution, and the American Museum of Natural History.
“It’s not that easy,” Marschall said. “You have to convince them that what you want to do is worth doing. He convinced them, as a student, without even the backing of an institution. I really appreciate Adam’s drive and motivation.”
Next came the measurement. Unlike chondrites, which are water-rich sedimentary rocks, Vesta and eucrites are made of basalt, much like the rock making up the seafloor.
Sarafian learned that WHOI geologist Nobu Shimizu had developed a technique to measure water trapped in glass pockets in basaltic seafloor rocks, using the Northeast National Ion Microprobe Facility at WHOI. Sarafian wanted to measure water in another mineral common in both seafloor rocks and meteorites: apatite. So he asked Shimizu and WHOI geologist Henry Dick if he could spend the summer at WHOI as a guest student working with them “to take the technique they already had and shape it toward measuring water in apatites.”
“A lot of people in the planetary field probably would have said, ‘You shouldn’t measure that—eucrites don’t have any water in them,’ ” Sarafian said. But for his master’s degree research at Georgia, he reported for the first time the presence of water in eucrites.
That feat inexorably led him to the next question: What is the source of the water? Which led to another high bar to hurdle: measuring hydrogen isotopes in extremely low concentrations of water.
Water, water everywhere
After his master’s degree, Sarafian naturally headed back to WHOI. While here, he met Marschall and another WHOI geologist, Sune Nielsen. They took him on as a guest student, for another summer and then a year, to continue his research while he applied to enter the MIT-WHOI Joint Program. The two are now Sarafian’s co-advisors for his Ph.D. research.
“We also have an awesome lab tech at the ion microprobe facility named Brian Monteleone,” Sarafian said, “and we began figuring out how we were going to do the measurements. Brian always says his favorite projects are where we’re pushing our machine to its very limits.”
There are two problems. First, “we’re measuring a minute amount of meteorite water, and we have to make sure we’re measuring nothing else. We’re constantly thinking that we do not want to be measuring any Earth water. And there’s water everywhere on Earth. We have to decontaminate the machine as best we can.”
They put the samples under a powerful vacuum for a week or two to suck away water. Then the samples go into the ion microprobe under ultrahigh-vacuum conditions that suck out virtually all air and water. Virtually all. The researchers still have to take meticulous measurements of extremely low concentrations of any residual hydrogen, calculating a baseline hydrogen standard in the machine and then subtracting it out of their final calculations.
The good news is that the mineral structure of apatite prevents Earthly water from diffusing in. So any water locked inside is meteoric. But rock surfaces can still have infinitesimal cracks that can trap infinitesimal amounts of terrestrial water.
“We polish the samples perfectly flat with the minimum of cracks possible, and that takes a lot of polishing,” he said.
Graduate student Adam Sarafian (left) works with his Ph.D. advisor, WHOI geologist Horst Marschall, in the Northeast National Ion Microprobe Facility at WHOI. (Jayne Doucette, WHOI)
Ready, aim, fire. Repeat.
The ion microprobe focuses a beam of ions on a very small area of a sample, about 10 microns wide by 10 microns long by 1 micron deep.
The beam sputters out ions from the sample, which are propelled through a mass spectrometer. It detects and distinguishes the ions based on their mass and charge—in this case, the ratio of normal to heavy hydrogen ions.
“We specifically analyze the cracks also, to know how much water might be in them and what its isotopic ratio is,” he said. “So it’s a lot of focusing, aligning, and re-running our standards, and if the beam is off by a little bit, it’s trouble. We’re running a lot of standards and running a lot of cracks. And each crack that we run takes an hour. Each standard we run is an hour, and every once in a while we get a data point. It’s painstaking, and we have to throw out quite a few analyses because we don’t think they are rigorous enough.”
In the end, the hydrogen isotope ratio in the eucrites looked just like Earth’s. “This means the water in the very early solar system, when eucrites were formed, was just like the water on Earth today.”
The study, published October 2014 in the prestigious journal Science, “shows that Earth’s water most likely
accreted at the same time as the rock forming the planet,” Marschall said. “The planet formed as a wet planet with water on the surface.”
“The answer is that our oceans were always here,” Sarafian said.
Graduate student Adam Sarafian (left) works with his Ph.D. advisor, WHOI geologist Horst Marschall, in the Northeast National Ion Microprobe Facility at WHOI. (Jayne Doucette, WHOI)
Reading and writing
Sarafian was lead author of the Science paper, with co-authors Nielsen, Marschall, Monteleone, and Francis McCubbin of the University of New Mexico Institute of Meteoritics. That made him recall his college days when reading a scientific paper was still a travail.
“Once I started focusing on geology, I said, ‘I really need to be able to read.’ There was a lot of re-reading papers, highlighting, notating on the side—hours and hours and hours of that. At first I just looked at the figures and the captions. I looked up a lot of words.
“I locked myself in my room or my office and just stayed up long hours rereading papers. I had a sleeping bag and a toaster oven in the office, and I was there quite a bit. I viewed that as me catching up to everybody else, because I felt so behind.
“It took a considerable amount of time and a lot of people helping to be able to read a scientific paper and get anything out of it. Eventually, I could read scientific papers quickly and thoroughly to get all the information out. But I still can’t really read fiction at all. I guess I am unwilling to put that much time into fiction.”
Traveling further back in time
Sarafian’s research road continues apace. He began analyzing samples of meteors that are 10 million years older than eucrites. These meteoritic bits that have occasionally fallen to Earth are called angrites, and they formed about four million years after the start of the solar system.
“If angrites have the same hydrogen isotopes as Vesta and Earth, it means water was accreting into these planetary bodies throughout the time they were forming, almost from time zero,” he said. He is also investigating if other elements that are essential for life to begin, such as carbon and nitrogen, may also have come along for the ride with hydrogen on carbonaceous chondrites and been delivered early to Earth.
Sarafian is now spending much of his time at the NASA-Johnson Space Center and at the Carnegie Institute at Washington, where he is using an ion probe facility that specializes in measuring extremely low amounts of hydrogen and carbon.
Meanwhile, somewhere out there, Vesta is still orbiting the far edges of our solar system, and an undergraduate is looking up the word “eucrite.”
This research is supported by a WHOI Mellon Award for Innovative Research, WHOI’s Ocean Venture Fund, and a NASA graduate fellowship.