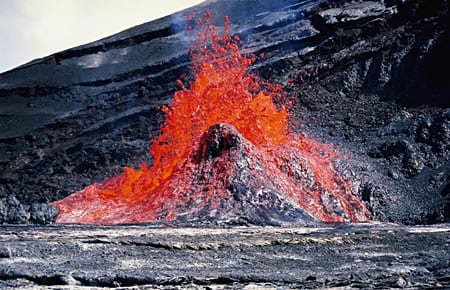
Conduits Into Earth’s Inaccessible Interior
Hot plumes surfacing from deep within the planet bring up telltale chemical clues about the mantle.
Jules Verne wrote about a way to journey to the center of the earth, but unfortunately, we haven’t found it yet. So we really don’t know what happens deep inside our planet.
Earth’s interior has yielded its secrets only gradually over the past few decades. Yet it contains evidence about how our planet originally formed some 4.6 billion years ago. And it holds clues to the processes that shape the surface of the earth—from the benign processes we covet (ore and mineral deposits) to the disruptive processes we yearn to predict and avoid (great earthquakes and volcanic eruptions).
We get glimpses of Earth’s interior from mountain-building processes that occasionally thrust rocks to the surface from depths as great as 95 kilometers (60 miles). Volcanic eruptions sometimes bring up rocks from depths of 200 kilometers (125 miles). But Earth’s rocky mantle continues down for another inaccessible 2,690 kilometers to the core-mantle boundary at 2,890 kilometers (1,800 miles). Nor will we ever directly view Earth’s metallic core, which extends another 3,481 kilometers (2,163 miles) down to Earth’s center.
Plumes that form islands
One way to probe the deep Earth is remote sensing, using seismic waves generated by earthquakes. By studying the varying speeds at which these waves travel through rocks, we can infer a great deal about the rocks’ chemical composition and their varying temperatures in different regions of the mantle. Still, the technique is indirect, and unfortunately, waves sometimes travel at similar velocity through rocks with different chemistry, providing ambiguous clues.
A more promising trail was blazed in 1972 when Jason Morgan proposed the “mantle plume” hypothesis. Mantle rocks are hot enough to be in slow but constant motion. But occasionally, unusually hot areas can form upwelling plumes. On the scale of the entire mantle, these plumes are narrow (with a width-to-height ratio similar to a 6-inch strand of spaghetti). But these plumes can be vast—plume “heads” can reach an estimated 500 to 1,000 kilometers (310 to 620 miles) in diameter.
Plumes start at the bottom of the earth’s mantle and carry material near to Earth’s surface—a trip that takes tens of millions of years. As these deep mantle rocks approach 50 miles from the surface, decreasing pressure allows them to partially melt. The melts, or magmas, may leak through the overlying cold rigid surface layer, or plate, to form volcanoes.
Most islands in the oceans are composed of volcanic rock derived from such plumes, as best we can tell. This seems like a promising avenue to reveal what lies at the bottom of the mantle. All we have to do is travel to island paradises and bring home volcanic rocks for analysis.
But, alas, it’s not that easy. Mantle rocks are chemically altered during the melting processes, and scientists have spent the last 20 years trying to reliably unravel these processes. Furthermore, only the mantle rocks that actually melted end up in labs—what’s left behind? And finally, we’re not sure all plumes come from the bottom of the mantle.
Where do plumes come from? Seismology provides our best way to detect plumes and map their sources, but the evidence is inconclusive. There are two schools of thought on the origins of plumes, the “layered mantle” school and the “whole mantle” school.
“Layerists” claim that the mantle has two layers. Below 660 kilometers (410 miles) deep, pressure and temperature conditions cause mineralogical changes in rocks. The deeper rocks are denser, and do not “flow” in the same way as shallower rocks. As a result, rocks above and below the 660-kilometer “boundary” convect separately. In this model, plumes arise solely from the bottom of the top layer. Virtually nothing from the deepest parts of the mantle ever comes near the surface.
“Wholists,” on the other hand, maintain that plumes come from the bottom of the mantle (1,800 miles deep), at the boundary of Earth’s core. Heat from the core disturbs the overlying mantle, leading to plume formation. If accurate, this model gives hope that we will someday understand the chemistry of the deepest mantle (and if we’re very lucky, of the outer core, provided some core material occasionally mixes into rising plumes).
“Fingerprinting” volcanic rocks
To explore the origin of plumes, geochemists analyze rocks from ocean volcanoes formed by plumes. They use a form of isotopic fingerprinting.
The rocks contain traces of long-lived radioactive isotopes left over from the initial building of our solar system. These elements have known half-lives—the time required for one-half of a quantity of “parent” isotopes to decay into more stable “daughter” isotopes.
We can calculate the rock’s age by measuring the relative amounts of remaining radioactive parent and its daughter products. And we can determine the “parent” rocks from which the specimen originally descended (or more accurately in this case, “ascended”!). In this way, we can trace a rock specimen’s lineage.
If the mantle convects in a single unit, it would produce volcanic rocks with a single homogeneous chemistry. A two-tiered mantle would produce, at most, two types of rocks. But, once again, the solution is not easy. Our analyses point to more chemical diversity in the mantle.
Pedigree and mongrel rocks
We have identified four “lineages” of plumes, and mixtures thereof. Some oceanic islands appear to be virtually pure species. Most are just mongrels.
The purebred ocean island rocks have been given particular labels. Rocks from Pitcairn Island, of HMS Bounty fame, are labeled “EM1” (enriched mantle 1). Rocks from Samoa, of Coming of Age fame, are “EM2.” Rocks from Mangaia, in the Cook/Austral chain, are called “HIMU” (High “mµ”, from the Greek symbol “µ,” which represents a uranium/lead ratio). Rocks from mid-ocean spreading ridges, which are fed from the shallow upper mantle, are “DMM” (Depleted Mid-ocean ridge basalt Mantle).
Note that even these purebred island rocks show some cross breeding. For example, specimens from Vailulu’u and Malumalu, two newly discovered undersea volcanoes in Samoa, show both the purest EM2 yet sampled, as well as a variety of less pure offspring. An unexplained curiosity is that all of the purebred islands are in the Southern Hemisphere.
Some of the well-known “mongrel” islands are Hawaii, Tahiti, and the Galápagos, Azores, and Canary Islands. Hawaii is notable for being both the largest of all mantle plumes (as measured by total volcanic output over the millennia) and by far the most intensively studied by geochemists
Recycled crust
The history of these purebred mantle rock species has been the subject of controversy for decades. Were they born when the earth was born, somehow escaping mixing in the constantly convecting mantle? Are they primordial, homogenous, and once-pristine mantle rock species that have been contaminated by injections of rock from Earth’s surface (as argued by Albrecht Hofmann and William M. White in 1982)?
The rigid plates of rock that cover Earth’s surface ultimately plunge back into the mantle—in the great subduction zones that border the Pacific Ocean and elsewhere. These plates carry ocean crust and its veneer of accumulated sediment into the mantle—sometimes deeply. This crust and sediment reintroduce chemicals into the mantle, which can confound our analyses.
For example, oceanic crust, during its millions of years of exposure to seawater, sequesters naturally radioactive uranium from the oceans. When this crust is subducted into the deep mantle, the uranium in it would decay, over a billion years, into significant accumulations of uranium’s daughter isotope, lead-206. This ancient oceanic crust material, heated up again in the deep mantle, might once again become buoyant—and thus a new plume is born! Rocks from this plume would have a high (and highly misleading) ratio of lead-206—the same fingerprint that typically distinguishes HIMU rocks.
Over millions of years, ocean crust also accumulates an overlying veneer of sediments which contain unusual amounts of rubidium, uranium, and lead from non-mantle sources. After subduction, hibernation in the mantle, and rejuvenation as a new plume, rocks containing recycled sediment material could contain “enriched” isotopic signatures that can confound our ability to trace the rocks’ ultimate source in the mantle.
The “recycling” proposed by Hofmann and White must occur, and it explains many aspects of the chemical “zoo” we observe in mantle rocks. Nevertheless, there is no unanimity whatsoever in the geochemical community as to whether this model, or any of its amended versions, is the “real” scenario.
Deep Earth odyssey
In 2004, here is the current state of our knowledge about the mantle. We know that Earth’s mantle is convecting, but we don’t know whether it is whole-mantle or layered convection. We are virtually certain that plumes exist, but don’t know with certainty where they originate. We know Earth’s mantle is not chemically homogeneous, but rather a chemical zoo with at least four purebred species and a bewildering pack of mongrels. We don’t know where any of these species come from, nor can we trace the pedigrees of the purebreds.
Given that it’s taken four decades of concerted effort to get this far, why are we optimistic and excited? Because in recent years, major links have formed among the fields of geochemistry, seismology, rock physics, and convection modeling. All these fields have also profited from the development of incredible new technologies that are allowing us to circumvent roadblocks that have stymied our scientific research for years.
Scientists must converse across disciplines more than ever. We need, each of us, to become broader—Renaissance scholars, as it were. We need to maintain access to cutting-edge technologies and continue to hone new ones. Then, possibly, an article on Earth’s mantle, written a decade from now, will contain the answers to the questions that linger in this one.
Slideshow
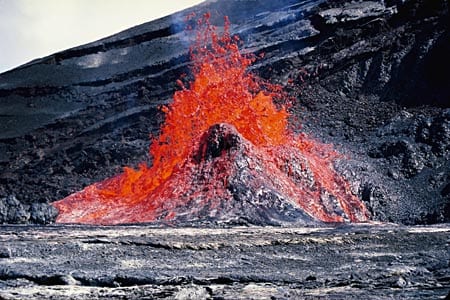
Slideshow
- A FOUNTAIN OF CLUES—Lava erupting on volcanic islands such as Hawaii is brought to the surface by narrow plumes of hot, buoyant rock originating in the mantle. The volcanic rocks contain chemical "fingerprints" that reveal their age, source, and formation—giving geochemists clues to the inner workings of the mantle.
- The underlying "flow"of materials in the mantle drives geological phenomena at Earth's surface, ranging from earthquakes and volcanoes to the creation of mountains and oceans.
- Shielding his face with an asbestos glove, WHOI geochemist Stan Hart uses a rock hammer to collect freshly erupted lava samples from Kilauea Volcano in Hawaii. The lava, orginating from Earth's mantle, provide chemical clues to the inner workings of the planet. (Albrect W. Hofmann.)
- By analyzing radioactive isotopes in rocks from various volcanic islands, geochemists have determined that all islands are not alike. Most islands are made of mixtures of many rock types. But some islands are primarily composed of one of four chemically distinct rock types: EM1 (Enriched Mantle 1), EM2 (Enriched Mantle 2), HIMU (HIgh "MU," from the Greek symbol µ); and DMM (Depleted Mid-ocean ridge basalt Mantle). These chemical distinctions provide clues to understand the underlying mantle plumes that create the islands. (Bruce Heezen and Marie Tharp. ? 1977)
Related Articles
- How did the ocean remain so quiet during Tonga’s eruption?
- Spock versus the volcano
- In Search of the Pink and White Terraces
- ROV Jason Images the Discovery of the Deepest Explosive Eruption on the Sea Floor
- Undersea Asphalt Volcanoes Discovered
- Deeply Submerged Volcanoes Blow Their Tops
- Robot Paints Stunning Map of Deep-sea Volcano
- Plumbing the Plume That Created Samoa
- New Wrinkles in the Fabric of the Seafloor