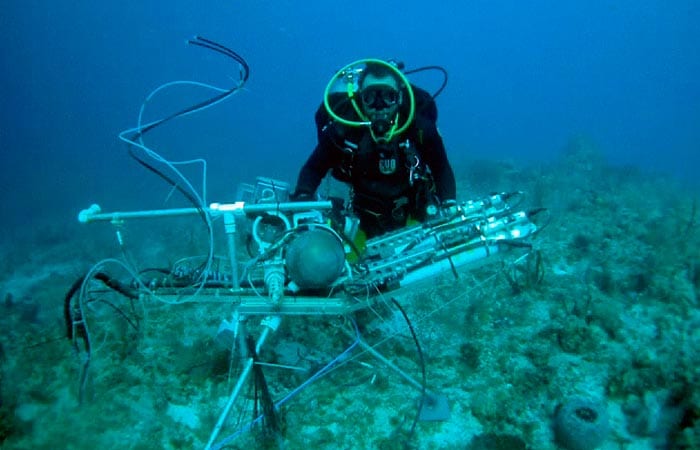
A Deep-sea Chemical-Sniffing Bloodhound
WHOI engineer develops a small, tough mass spectrometer to work in the ocean
<!–
–>
Researchers can learn complicated things from some of the simplest animals in the ocean. Case in point: Rich Camilli’s work on sponges near Aquarius, an undersea laboratory 63 feet below the ocean surface in the Florida Keys National Marine Sanctuary. Camilli, an assistant scientist at Woods Hole Oceanographic Institution (WHOI), recently spent 10 days in the submerged lab, which is operated by the National Oceanic and Atmospheric Administration. Oceanus magazine contributor Matt Villano caught up with him shortly after he returned in September 2008.
What specifically was your mission and research on Aquarius?
I was there to operate an instrument called a TETHYS mass spectrometer. It’s an in situ underwater mass spectrometer that I designed and built in partnership with Monitor Instruments Co. In general, mass spectrometers identify and measure the composition of chemical compounds. In this case, we’re using the mass spectrometer to monitor and investigate biochemical pathways in sponges, in which they’re using nitrogen and converting it from one type of molecule to another.
Hold on, let’s back up a minute. I envisioned mass spectrometers as big machines that fill up special rooms in labs. What did you have to do to make one that works under the sea?
I guess one engineering challenge was to make the TETHYS instrument small. It’s about the size of a watermelon. Most mass spectrometers are much larger than that—one on WHOI’s Quissett campus is the size of an entire building. This is one of the smallest mass spectrometer designs in the world, and it’s also one of the lowest-powered instruments. It takes about 25 watts. That’s means the device requires less power than the light in your refrigerator.
Mass spectrometers generally like to work in laboratories, because they’re designed to operate in controlled environments—where there’s temperature control, where they’re not subject to vibration, or electronic interference, that kind of thing. The TETHYS instrument is designed to operate in these kinds of extreme environments—under high pressure, under vibration, under all kinds of temperatures. These are challenging from a design perspective. The other challenges are data storage and maintaining calibration. The instrument has to maintain its calibration while it’s taking measurements. The TETHYS design is truly autonomous. You can just provide it power, and it takes care of all of that.
So you can bring the mass spectrometer to a sample under water, instead of vice versa. What are the advantages of that?
First off, it’s the quantity of data that you get. In the time that we were down on the bottom, we collected on the order of 10,000 measurements. You can imagine what 10,000 water samples would look like if you had to stack them up. The other problem is that transporting samples introduces the issue of transformation. These are bioactive compounds that we’re looking at, bioactive gases. When you take a sample and then take it up to the surface, its environmental conditions change. The pressure changes, the temperature changes, and typically you expose it to light and other things that may cause a chemical transformation. If you don’t measure instantly what these organisms are doing right there in seawater, the quality of your measurements could suffer.
What are the some of the other challenges of using a mass spectrometer under water?
One challenge is that mass spectrometers require a near-perfect vacuum. Typically, inside the instrument, we have pressures down between a millionth and a billionth of an atmosphere. (One atmosphere is what we experience on Earth’s surface). Also, mass spectrometers typically hate water. They can’t get any water inside of them. So we have to allow compounds to come in, but we cannot let in any water. Basically, we tend to use membranes to do that. It’s not unlike the way a desalinization system works using reverse osmosis.
One thing that was kind of a pleasant shock to me was that when we were doing these experiments, we were using enclosures around the sponges, so that they didn’t have access to a continuous supply of ambient seawater. We put them in an enclosure where there was a limited volume of water, and they were re-circulating it through their tissues. Well, one of the things the mass spectrometer picked up was that the enclosure material itself was giving off solvents into the water. We managed to track the solvents down to the adhesive from a few pieces of tape in the enclosures. Obviously, we had to go back and solve that problem. But, it was great that we could see this interference while we were working and knew how to fix it.
How accurate is the underwater device?
I spent many years working on the design of these instruments and, typically, we can measure chemicals at concentrations down to less than a part per billion. We can also do in situ measurements of not just molecules, but specific isotopes of elements. The isotopes tell you a great deal about the origin of that chemical.
So, for example, in our experiments near Aquarius, we were dosing the sponges with a particular kind of isotope—an ammonium chloride isotope containing a nitrogen atom with an extra neutron. And we were using the TETHYS instrument to track that isotope and watch it be transformed by the sponge into the nitrogen gas.
What did you find?
We found evidence of a particular kind of sponge that takes ammonia from the ocean and converts it into nitrogen gas through a multi-step process. This process is called “Anamox.” It was discovered maybe a decade ago, but it was thought that only microbes living in the water column or ocean sediments could do it. With our research, we’ve found evidence that the process also is happening in these sponges. It has big implications for how nitrogen cycles through the ocean ecosystem.
What are those implications?
Basically, these findings may suggest that these sponges have a competitive advantage in terms of their ability to use ammonia where other organisms do not. Down there on Conch Reef in Florida, particularly over the past 20 years or so, there’s been a rapid shift in the reef ecosystems—the corals are dying off, and the sponges are becoming more numerous. So it may be that the abundance of various nitrogenous compounds in the ocean there—perhaps from increasing fertilizer runoff—is allowing sponges and these other organisms to out-compete the corals.
What more can you tell us about the sponges?
Well, for example, one variety of sponges is known as “stinker sponges.” If you were to cut a piece out of one of these and bring it up to the surface, the piece would have a pretty foul odor. But that smell provides a pretty good indicator of the biochemistry that’s going on there, and a good indicator that this sponge is unlike other sponges. There’s also some evidence that these organisms smell because they are engaging in sort of chemical warfare to fend off predators.
Were you looking at other aspects of sponge life with the mass spectrometer?
The other thing that we were looking at was the oxygen consumption and CO2 (carbon dioxide) production by the sponges. They engage in aerobic respiration just like you or me, but they have huge, huge pumping rates and very high metabolic rates. Sometimes they turn on and pump really hard, and sometimes they just shut off. Nobody really knows why. So they’re a lot more complicated in terms of their biochemical functioning than we tend to appreciate, but particularly in terms of the CO2 production. That has huge implications for the acidification of the reef. Increasing CO2 dissolved in seawater generally yields increasing carbonic acid, and that can make it harder for corals to build their skeletons.
Now that you’ve completed this 10-day research trip, what’s next?
There was a follow-up mission in mid-October. I couldn’t be there because I was on an expedition in the Pacific, but one of my brothers is a diver and also an oceanographer, so he took my place. Overall, the ultimate goal of these projects is to identify if sponges are capable of denitrification [turning ammonia into nitrogen gas], and if so, then identify the biochemical pathways, and to look at this issue of the acidification of the reef caused by organisms like the sponges that are producing CO2. Our work down there really has just begun.
Have you used your underwater mass spectrometer for other kinds of research?
Yes. Mass spectrometry has broad application in oceanography, so I’ve used these instruments in a range of programs.
An experience that I will probably remember for many years was the first time that I used a mass spectrometer on a human-occupied submersible to study an underwater volcano in the Aegean Sea. It was the first time anyone did this, so the technological complexity was daunting. During the initial descent I was on pins and needles, monitoring equipment status while I went through mental checklists trying to anticipate any problem before it arose. But as we reached the flank of the volcano and the data began rolling in, I had one of those Zen moments. We could use the mass spectrometer’s readings to guide us through the darkness right to one of the volcano’s active venting sources.
Following Hurricane Katrina, I also began using mass spectrometers to help various federal agencies with oil spill cleanup efforts in the Gulf of Mexico. That work was a great success, and now my group routinely gets called to assist when there is a spill or disaster. Over the past three years, we have developed new techniques that can pinpoint leaks among infrastructure debris and map contaminated seafloor sediments in real time.
On my recent Pacific expedition, we equipped TETHYS mass spectrometers on seafloor landers developed by the German ocean research institute IFM-Geomar. These robotic landers are roughly the same size and shape as the Apollo moon lander. They’re designed to collect samples and conduct incubation experiments for several days at a time, until they hear an acoustic command from the ship to return to the surface.
The expedition was an international collaboration with the Government of Peru, IFM-Geomar, and WHOI’s Reinhart Access to the Sea program. It studied an area off the coast of Peru, which is one of the most biologically productive areas in all of the world’s oceans. But during certain times of the year, it becomes anoxic (depleted of oxygen). Not much is known about how the benthic organisms adjust to these seasonal changes or other longer-term ocean and climate patterns affecting the area, such as the El Niño/La Niña cycles. The TETHYS mass spectrometers will supply data to analyze biochemical activity of organisms in this so-called Oxygen Minima Zone, or OMZ.
Development of the TETHYS mass spectrometer was supported by a research grant from the National Ocean Partnership Program.
Video
Featured Researchers
See Also
- A Robot Starts to Make Decisions on its Own from Oceanus magazine