Back to WHOI Arctic Group Projects |
|
MARINE POLLUTION BULLETIN,
VOL. 43, NO. 1-6, PAGES 61-70, 2001
Two Regimes of the Arctic's Circulation from Ocean Models with
Ice and Contaminants [PDF
version]
Andrey Proshutinsky
Woods Hole Oceanographic Institution, USA.
Mark Johnson
Institute of Marine Science, University of Alaska, Fairbanks, AK, USA.
Note that this is a condensed version of the article. The full (PDF
format) version is also available (click "PDF version" above).
|
|
Abstract:
A two-dimensional barotropic, coupled, ocean-ice model with a space
resolution of 55.5 km and driven by atmospheric forces, river run-off, and
sea-level slope between the Pacific and the Arctic Oceans, has been used to simulate
the vertically averaged currents and ice drift in the Arctic Ocean.
Results from 43 years of numerical simulations of water and ice motions demonstrate that two
wind-driven circulation regimes are possible in the Arctic, a cyclonic and
an anti-cyclonic circulation. These two regimes appear to alternate at 5-7 year
intervals with the 10-15 year period. It is important to pollution
studies to understand which circulation regime prevails at any time. It is anticipated that 1995 is a year with
a cyclonic regime, and during this cyclonic phase and possibly during past
cyclonic regimes as well, pollutants may reach the Alaskan shelf. The regime shifts
demonstrated in this paper are fundamentally important to understanding
the Arctic's general circulation and particularly important for estimating pollution
transport.
1. Introduction

The variability of the general circulation of the Arctic is not as well known
as it is in many other oceans. Knowledge of the circulation is important to
understanding the potential for pollutants and radionuclides to be carried to locations
distant from a source. The thrust of the present work is to examine the major
forcing mechanisms that drive the Arctic's circulation, and with the use of a basin
scale model, identify the relative strengths of each forcing mechanism, and the
Arctic's response. The potential for pollutants to be dispersed depends on the total
circulation comprised of the flow resulting from each forcing mechanism.
Two different historical views describe factors determining the movement of
water and ice in the Arctic Ocean. Shokal'skii (1940),
Gordienko and Karelin (1945), and Buinitskii, 1951 and
Buinitskii, 1958 assumed that the surface
circulation in the Arctic Ocean was mainly driven by the inflow of Atlantic
water through Fram Strait, producing an excess of water in the basin and causing an outflow of
surface water and ice along the shorter routes to the Greenland Sea. Data from
vessel drifts in the Eurasian subbasin indicated a movement of surface water from
the Eurasin subbasin to Fram Strait. Nansen (1902), who discovered waters of
Atlantic origin in the Arctic Ocean, mentioned that water exchange between the Arctic
Ocean and the Greenland and Norwegian Seas was caused by differences of water
temperature and salinity, e.g., he assumed a thermohaline circulation. These
scientists believed the Arctic Ocean water and ice circulation was mainly
thermohaline in nature and closely related to the world's ocean circulation.
Subsequently, some authorities (Zubov and Somov, 1940;
Shuleikin, 1941;
Shirshov, 1944; Treshnikov, 1959) adopted a different view on the nature of the
water circulation, namely, that the inflow of Atlantic waters into the Arctic Basin
was caused principally by the outflow of surface water to the Greenland Sea
under the influence of the existing wind system. This point of view was confirmed by
experiments undertaken by Gudkovich and Nikiforov (1965) with a hydraulic model
simulating the system of wind-driven currents in the Arctic Ocean. The
experiments showed winds to be a principal factor in forming the system of
permanent currents in the Arctic Basin. The winds of the Arctic anti-cyclone generate
anti-cyclonic currents in the Amerasian subbasin. The wind system in the
Eurasian subbasin and at the periphery of the Arctic anti-cyclone induces a wide
Trans-Arctic current originating in the Chukchi Sea and emerging through Fram
Strait. Simulating the inflow of water as the only factor driving the circulation did
not produce a circulation pattern similar to that actually observed. In
accordance with this concept, Gudkovich, 1961a and Gudkovich, 1961b explained the seasonal and
year-to-year variation in the circulation of surface water and ice by a suitable
rearrangement of the baric fields.
To confirm Gudkovich's conclusions, a series of two-dimensional numerical
models were run (Fel'zenbaum, 1958; Campbell,
1965; Galt, 1973; Ponomarev and
Fel'zenbaum, 1975; Proshutinsky, 1988 and Proshutinsky,
1993), where wind
fields were the major factor driving circulation in the Arctic Ocean. It is
interesting that Galt (1973) found that inflow/outflow forcing and bottom topography both
play a more important role in the circulation than does wind forcing. However,
in the research of Treshnikov and Baranov (1972), it was demonstrated that the major
features of the water circulation may be obtained by means of diagnostic
calculations according to density fields. These authors stated that "we could
not evaluate the role of individual factors in the formation of currents because
the observed field of masses already contains the combined (and still
indistinguishable) effects of wind, baroclinicity, and bottom relief". A similar
conclusion could be made according to the results of the diagnostic model by Ponomarev
(1977).
Prognostic models (Semtner, 1976) testify to the significant role of the
irregularity of the field of mass in the formation of the three-dimensional water
circulation.
A series of different model runs was presented by Semtner
(1987), Fleming and
Semtner (1991), Häkkinen and Mellor (1992), Hibler and Bryan
(1986), Riedlinger and Preller (1991), Piacsek et al.
(1991). All of these models and numerical
experiments were directed to developing new models and to describing better
observed features of water and ice dynamics without special attention to the
variability of the oceanographic regime of the Arctic Ocean. Recent papers by
Häkkinen, 1993 and Häkkinen, 1995 were dedicated to modeling and explain such phenomena as
deep convection in the Greenland Sea and the Great Salinity anomaly. Her
investigations demonstrated again the significance of atmospheric circulation
in the coupled ice-ocean system in the Arctic.
In this paper we assume that the general circulation of the Arctic can be
characterized by four broad responses to different forcings. The response from
the observed sea-level slope between the North Pacific ocean and the Arctic is northward
flow through Bering Strait and out through Fram Strait, with the interior
response yet to be clearly described. A second response is due to forcing by the atmospheric
winds and pressure fields that drive both a baroclinic and a barotropic
response. In this paper we treat the Arctic as a barotropic fluid. A third response is due to
the inflow from rivers. Associated with river inflow is a fourth response, the
total thermohaline flow, due to the introduction of fluid at a boundary.
We have modeled the response due to sea-level slope, winds and river input.
The relative strength of the circulation regimes driven by these forces can be
compared by examining the model results. A comparison with the thermohaline flow is
possible by comparing the results of modeled thermohaline flow by Proshutinsky,
1988 and Proshutinsky, 1993 or Polyakov
(1994). Such a comparison shows that the
mean annual wind-driven circulation in the Arctic is at least the same order of
magnitude or larger, in terms of maximum observed current speeds, as the
thermohaline forcing. Thus, the circulation regimes presented in this study may
be observable in the ocean, depending on the Arctic's linearity.
For this paper we have examined the way the circulation in the Arctic
responds due to the sea-level slope, the winds (derived from pressure), and the
input from rivers. We identify two wind-driven regimes, a cyclonic and an anti-cyclonic
regime, which alternate over a ten to fifteen year period. When estimating
pathways that pollutants may follow, it is important to distinguish between these two
regimes, and identify which phase is present.
That the position of the sea surface may serve as an integral index of
processes of interaction between the atmosphere and the ocean was an idea first
stated by Duvanin (1951). This result was confirmed later by different authors
depending on the problem they solved, e.g., "the position of the sea surface is
an integral indicator of most processes and phenomena related to the formation of the
hydrological regime" (Galerkin, 1961), or "the only indicator of synoptic
variability of hydrological conditions in arctic seas, for which corresponding field data
exist, consists of the sea-level heights" (Krutskikh, 1978). Year-to-year and
seasonal fluctuations of the ocean level in this sense may be viewed as an indicator
of large-scale interaction between the atmosphere and ocean. In particular, the
sea surface can evidently be used as an indicator of large-scale circulation of water and
ice of the Arctic Ocean and as an indicator of the regime driving pollution
transport. We use sea level in our paper as an integral index to describe variability of
the Arctic Ocean circulation.
This paper is organized in the following manner. Basic equations and boundary
conditions are described in Model Equations and Boundary Conditions (PDF version only). The data
base is discussed in Data Base. The model results are given in Results and
the final section contains a summary and discussion.
2. Data base

To cover the Arctic Ocean with a 55.5 km spatial grid step we use bathymetry of the Arctic Ocean with a 14-km spatial grid step prepared by
Kowalik and
Proshutinsky (1994).
The atmospheric forcing fields are obtained from the CD-ROM of the National Center for Atmospheric Research (NCAR). We use sea-level pressure analysis for
the daily wind forcing case (NCAR, 1990).
The model results are supported by comparing observations to information obtained by different authors in numerical experiments with the Arctic Ocean models.
Three kinds of data bases are available to perform this comparison:
(a) Sea-level observations are available from about 15 sites in the Kara, Laptev, East-Siberian and Chukchi Seas. These are mainly statistical characteristics of
sea-level regime which were published mainly in Russian scientific journals.
(b) Coordinates of 335 drifted buoys for the period 1979-1988 (Throndike et al.,
1982).
(c) We use widely different indirect information related to the hydrological regime of the Arctic Ocean, such as dynamic heights relative to the 200 dbar surface
obtained by Treshnikov et al. (1976) for the period 1946-1965; water temperature and salinity record in the Faroe-Shetland Canyon for a period of 1946-1973
published by Yanes (1977), air temperature record at the meteorological stations in the Greenland, Norwegian and Barents Seas, published by
Dementiev (1991),
and water transport, salinity and temperature behavior in the Fram Strait simulated by
Häkkinen (1995).
3. Results:

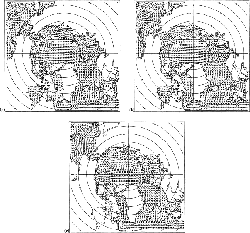 |
 |
Fig 1. Surface currents
from the barotropic model with prescribed: (a) river run-off
and sea slope between the Pacific and Arctic Oceans; (b) only
sea slope between the Pacific and Arctic Oceans; (c) only river
run-off. Note that with river run-off forcing flow through Bering
Strait is southward. [Larger image]
|  |
Numerical simulations have been completed to assess
the effects of different forcing. In the first experiment we tried
to understand the role of Bering Strait inflow and a river run-off
in the Arctic Ocean circulation. Antonov,
1958 and Antonov, 1968 had explained
observed surface circulation and the Arctic Ocean hydrological regime
mainly by river run-off variability.
Fig. 1(a) shows the surface circulation in the Arctic Ocean
for the first experiment. The sea slope between the Pacific and
the Arctic Oceans, and the river run-off, drive the well-known,
quasi-permanent circulation in the northern Bering Sea, Bering Strait,
Chukchi Sea, Laptev and Kara Seas. They also indicate the location
of the core of the Trans-Arctic and East-Greenland currents. Magnitudes
of the currents are proportionally related to the values of sea-level
slope between the Pacific and Arctic Oceans and the river run-off.
The general pattern of these currents is related to the ratio between
water transport through the Bering strait and the water inflow from
the Siberian rivers. Two extreme situations are shown in
Fig. 1(b) and (c), where surface circulation is presented for
situations when sea slope is zero and only river run-off forms the
circulation, and when river run-off is zero and circulation in the
ocean is driven only by sea slope between the two oceans.
In the second experiment, both the ocean and ice models were initialized from rest on 1 January 1946, and run for 43 years until 31 December 1988, using the
NCAR daily surface atmospheric pressure, the simulated surface winds computed from the pressure data, mean annual river run-off and a sea-level slope between
the Pacific and Arctic Ocean which provided reasonable inflow of Pacific waters of about 1 Sv. Barotropic model spin up takes about 4 months if sea-level slope
and river run-off are included into the model.
|
4. Results: Year-to-year variability

 |

Fig. 2. Surface currents and surface
atmospheric pressure distribution for the typical year with
anti-cyclonic circulation. [Larger image]
|
 |
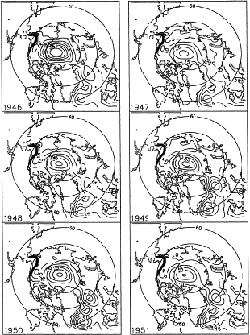
Fig. 3. Sea-level heights for
the years of anti-cyclonic circulation. High heights are in
the center of the Arctic Basin and low heights are along the
coast. There is a cyclonic circulations in the Greenland-Islandic-Norwegian
Seas (GIN) and in the North Atlantic. [Larger image]
|
 |

Fig. 4. Surface currents and surface
atmospheric pressure distribution for the typical year with
cyclonic circulation. [Larger image]
|
 |
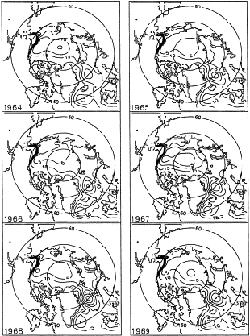
Fig. 5. Sea-level heights for
the years of cyclonic circulation. Low heights are in the
center of the Arctic Basin and high heights are along the
coast. There is a cyclonic circulations in the Greenland-Islandic-Norwegian
Seas (GIN) and in the North Atlantic. [Larger image]
|
The model shows that wind is a principal factor responsible for the year-to-year variability in the circulation of ice and vertically integrated flow. The time for the
ocean to respond to changes in thermohaline forcing is generally considered to be too slow to explain shorter term variability of a few years or less.
Two regimes describe the modeled barotropic circulation and ice
circulation in the Arctic Ocean. One regime is characterized by
anti-cyclonic circulation as observed in the modeled central Arctic
Ocean during 1946-1952, 1958-1964, 1972-1979 (Fig.
2 and Fig. 3). A second
regime is characterized by cyclonic circulation as observed in the
model during 1952-1957, 1964-1971 and 1980-1986 (Fig.
4 and Fig. 5). Regime
shifts between cyclonic and anti-cyclonic flow occur every 5-8 years
with the period of oscillation of about 10-15 years.
To determine the time scale of the regime shifts we examined the
sea level slope at the center of the Arctic Basin as a measure of
cyclonicity. The sea slope was calculated as the difference between
the sea level at the center of a closed circulation cell and the
sea level at the periphery of this circulation divided by the distance
between the center and periphery. Positive slopes corresponds to
a raised sea surface and anti-cyclonic ice and water circulation
(Fig. 3). Negative sea-level
slopes in the center of the Arctic Basin correspond to cyclonic
ice and water circulation (Fig.
5). The absolute value of the sea-level gradient in the center
of the Arctic Basin may serve as an index of circulation intensity
(higher values correspond to a higher velocities in cyclonic or
anti-cyclonic circulation).
Fig. 6 shows a time-series of the gradient of this slope and
identifies the circulation and strength of the two regimes.
We have found references to the existence of only a 5-7 year oscillatory
regimes in the Russian meteorological and oceanographic literature.
This is probably because the time series of analyzed data were too
short. Karklin (1977) found that the ice
conditions and ice drift in the Arctic Seas has a periodicity of
6-7-years. A 7-8-year period was found in the temporal variability
of dynamical heights relative to 200 dbar for the period 1949-1973
(Treshnikov et al., 1976). An investigation
of the Arctic Ocean water budget (Treshnikov
and Baranov, 1976) revealed a 2-3 and a 5-7 year period. Our
results show that 2-3 year and a 5-7 year variability of the circulation
exists, too, on a background of cyclonic or anti-cyclonic circulation
(this is usually 2 year variability of intensity of the circulation
without changing the sign of rotation). Year-to-year variability
of temperature and salinity in the Faroe-Shetland Canyon shows periods
of 5-7 years as well (Yanes, 1977). Air surface
temperature at the stations in the Greenland and Norwegian Seas
has the same period 5-7 year oscillations (Dement'ev,
1991). It is interesting that in the most recent paper of
Häkkinen (1995), one can find the same 10-15 years variability
in the simulated potential temperature and salinity for the top
100 m, and in the change in heat and salt content of the upper 2000
m in the Greenland Sea on her
Fig. 6 and Fig. 7(a),
respectively.
|
Fig. 6. Gradients of sea level
in the central Arctic Basin. Positive gradients indicate anti-cyclonic
circulation and negative gradients indicate cyclonic circulation.
In 1995-1999 we expect a cyclonic regime to prevail. [Larger image]
|
Fig. 7. Trajectories of water
parcels released at different regions in 1946. Period of simulations
is 1946-1988. [Larger image]
|  |
The presence of two wind-driven circulation regimes has several important implications. During years of anti-cyclonic ice and water circulation, the core of the
Trans-Arctic current is intensified and shifted toward Siberia, enhancing outflow of ice from the East-Siberian, Laptev and Kara Seas. The ice transported through
Fram Strait is expected to be thinner because of its origin in these coastal seas, implying decreased transport of fresh water into the Greenland Sea. During years
with cyclonic circulation, the core of the Trans-Arctic current is shifted toward Canada and Greenland, enhancing the flow of old ice into the Greenland Sea.
According to this ice circulation, there should be more transport of ice and fresh water through Fram Strait at this time because the ice transported from the
Canadian sector of the Arctic is thicker than in the Siberian Seas by a factor of 2 or more. Navigation in the Kara Sea is likely to be better in the years with
anti-cyclonic type of circulation and worse in the cyclonic years when ice tends to block the Vilkitskogo Strait.
|
5. Results: The annual cycle

After analyzing the "average" annual cycle we have found that the spatial-temporal structure of the surface heights of the Arctic Ocean is determined mainly by the
surface atmospheric circulation: by the intensity and location of the Arctic anti-cyclone and Icelandic depression. In the cold season from November to April there is
significant development of high pressure in the Arctic with a rise in the sea level in the center of the anti-cyclonic system of circulation and a decrease in sea level
along the coast. In the second half of the year with a weakening of the arctic atmospheric anti-cyclone, the resulting barotropic anti-cyclonic ocean circulation
weakens, with sea level in the center of circulation dropping while there is a sea level increase along the coastline of the arctic seas.
In the Norwegian and Greenland Seas, under influence of the system of winds and the baric field of the Icelandic depression from November to April, a vast
cyclonic water circulation develops. In the warm season the atmospheric depression fills and wind-driven circulation weakens.
The annual cycle has been examined with respect to the two general wind-driven circulation regimes. We have averaged into monthly bins data from the anti-cyclonic
circulation regime and averaged into monthly bins data from the cyclonic circulation regime for ice and surface motions to look at the seasonal cycle of each of the
two circulation regimes. In the anti-cyclonic regime, strong anti-cyclonic circulation dominates for about 10 months from October to July. Thus, in this case, the
winter regime of annual circulation prevails. In the years with a cyclonic regime, the summer circulation, characterized by weak cyclonic circulation and low ice and
water transport through the Fram Strait, dominates the annual cycle.
6. Results: Pollutant transport

Understanding the two circulation regimes is useful for investigating the temporal
and spatial variability of ice, water and pollutant transport in
the Arctic Ocean. For example, in
Fig. 7 we present results based on the history of trajectories
of water markers released monthly beginning in 1946 at Bering Strait
and at different river mouth locations for the period 1946-1986.
Water markers moving with vertically averaged velocities have comparatively
stable trajectories following bathymetric features and are consistent
with the two circulation regimes. For example, parcels released
at Bering Strait have trajectories with both cyclonic and anti-cyclonic
rotation. Eighty percent reach Fram Strait and 20% were entrained
into the circulation of the central Arctic Basin. Parcels originating
in the Kolyma River mouth move to the east or west as a function
of the regime type. Pollutants from the Kolymya River can reach
the coastal waters off Alaska.
Ice trajectories are more variable because of the direct influence of wind. Tracks of the ice motion can be found everywhere in the Arctic basin with a maximum
concentration in the Beaufort Sea and along the core of the Trans-Arctic Current.
 |
Fig. 8. Conditional concentration
of pollution from the different regions during 1946-1988.
Note higher concentrations stretching from the Alaskan shelf
across the Arctic Basin to Fram Strait; the higher concentrations
in the Beaufort Gyre and along the east Siberian shelf. [Larger image]
|
Fig. 8 shows concentration of pollutants
from different sources. Pollutant concentration was simulated using
water and ice trajectories, and we assumed that at every time step
each "pollutant particle" leaves an amount of its pollution
that is simply summed every model time step. Summing gives a `concentration'
and a contour of this is presented. To have a high concentration of
pollution in a region either, (1) many particles pass over the region,
or, (2) a particle remains in the region for an extended period. Analysis
of the figures shows that there are a few regions with high potential
pollutant concentration: the continental slope of the Siberian Seas,
the core of Trans-Arctic current, regions with prevailing local cyclonic
circulation, and, of course, regions near a source of pollution.
7. Summary and Conclusions

We have constructed a barotropic, two-dimensional, coupled, ice-ocean model with resolution of about 55 km, obtained solutions to different kinds of forcing, and
analyzed data of vertically averaged currents and ice motion in the Arctic Ocean for period 1946-1988. The model results show that:
1. Two wind-driven circulation regimes are possible in the Arctic, a cyclonic and an anti-cyclonic circulation. These appear to alternate at intervals of 5-7 years with
the period of 10-15 years. It is important to pollution studies to understand which circulation regime prevails. It is anticipated that we are now in a cyclonic regime,
and during this phase, pollutants can indeed reach the Alaskan shelf.
2. Many measurements of temperature and salinity distribution were done in the Arctic Ocean between 1972 and 1978 when an anti-cyclonic circulation regime
prevailed. These data were used in forming the Levitus data base as well as for modeling the thermohaline circulation of the Arctic Ocean. Our knowledge about the
Arctic Ocean circulation is thus based primarily on data from years with prevailing anti-cyclonic atmospheric and ocean circulation. That a cyclonic circulation regime
also exists is supported by examining the temporal variability of dynamic heights in the Arctic Ocean (Treshnikov et al.,
1976), temperature and salinity oscillations in
the Faroe-Shetland Strait (Yanes, 1977), and air temperature variations in the Norwegian and Greenland Seas (Dement'ev,
1991).
3. The seasonal circulation has two regimes as well. During winter an anti-cyclonic regime prevails and during summer this anti-cyclonic circulation weakens or
perhaps even reverses so that weak cyclonic circulation prevails.
4. We anticipate that this research will continue, and we will include the atmospheric conditions for 1989-1994. Following this, we plan to use a three-dimensional
model for simulating the Arctic Ocean circulation including the thermohaline effects and ice thermodynamics and further explore the two circulation regimes discussed
here.
The regime shifts demonstrated in this paper are fundamentally important to understanding the Arctic's general circulation and particularly for estimating pollution
transport. But because the majority of Arctic data reflect the anti-cyclonic (1972-1978) regime, and because the recent Trans-Arctic effort may have sampled
during an anti-cyclonic regime as well, it is central to Arctic science that this modeling work be fully tested and a major future field effort sample during the next
cyclonic regime (1995-1999).
8. Acknowledgements

We would like to acknowledge support for this work from the Office of Naval Research, under grant NOO14-94-1-0570. Numerical computations were carried
out using CRAY Y-MP located at the University of Alaska, Fairbanks.
9. References

- Antonov, V. S. (1958)
- Role of river run off in the regime of the Arctic Ocean circulation. Problemy Severa 1, 52-64.
- Antonov, V. S. (1968)
- Nature of the ice and water motion in the Arctic Ocean. Trudy AANII 285, 148-177.
- Ashik, I. M., Proshutinsky, A. Yu. and Stepanov, V. A. (1989)
- Some results and prospects of numerical forecasts of storm surges in Arctic Seas. Meteorologiya i
Gidrologiya (Soviet Meteorology and Hydrology) 8, translated from Russian by Allerton Press, pp. 74-82.
- Buinitskii, V. Kh. (1951)
- Formation and drift of ice cover in the Arctic Basin. In Trudy dreifuyushechei ekspeditsii Glavsevmorputi ns l/p G. Sedov, vol. 4,
Moskva-Leningrad, pp. 12-40.
- Buinitskii, V. Kh. (1958)
- Perennial flow of surface water and the general pattern of sea ice drift in the Arctic Basin. In Nauchnye doklady vyshei shkoly.
Geologichecko-geograficheskie nauki, vol. 1, pp. 17-31.
- Campbell, W. J. (1965)
- Wind-driven circulation of ice and water in a Polar Ocean. Journal of Geophysical Research 70 (14), 3279-3303.
- Dement'ev, A. A. (1991)
- Year-to-year oscillations of air temperature in the Greenland and Norwegian Seas. Problemy Arktiki and Antarktiki 65, 6-13.
- Duvanin, A. I. (1951)
- Average level of oceans as an index of processes of interaction of the atmosphere and the ocean. Trudy GOIN 9, 16-74.
- Galerkin, L. I. (1961)
- Problems related to research of the seasonal oscillations of the World Ocean level. Okeanologiya 1 (6), 1108-1117.
- Galt, J. A. (1973)
- Numerical Investigation of the Arctic Ocean Dynamics. Journal Physics and Oceanography 3 (4), 379-396. INSPEC
- Gordienko, P. A. and Karelin, D. B. (1945)
- Problems of the motion and distribution of ice in the Arctic Basin. Problemy Arktiki 3, 25-38.
- Gudkovich, Z. M. (1961a)
- Relation of the ice drift in the Arctic Basin to ice conditions in Soviet Arctic seas. Trudy okeanographicheskoi komissii 11, 14-21.
- Gudkovich, Z. M. (1961b)
- On the nature of Pacific currents in the Bering Strait and the causes of seasonal variations in their intensity. Okeanologiya 1 (4),
608-612.
- Gudkovich, Z. M. and Nikiforov, E. G. (1965)
- Investigation of water circulation in the Arctic Basin using a model. Okeanologiya 5 (1), 75-83.
- Fel'zenbaum, A. I. (1958)
- Theory of steady-state ice drift and calculation of multi-year averaged ice drift in the Central Arctic Basin. Problemy Severa 2, 16-47.
- Fleming, G. H. and Semtner, A. J. (1991)
- A numerical study of interannual ocean forcing on Arctic ice. Journal of Geophysical Research 96, 4589-4604.
- Häkkinen, S. (1993)
- An Arctic source for the Great Salinity anomaly: a simulation of the Arctic ice ocean system for 1955-1975. Journal of Geophysical
Research 98 (16), 397-16,410.
- Häkkinen, S. (1995)
- Simulated interannual variability of the Greenland Sea deep water formation and its connection to surface forcing. Journal of Geophysical
Research 100, 4761-4770. GEOBASE INSPEC OceanBase
- Häkkinen, S. and Mellor, G. L. (1992)
- Modeling the seasonal variability of the coupled Arctic ice-ocean system. Journal of Geophysical Research 97 (20),
285-20,304.
- Hibler, W. D., III and Bryan K. (1986)
- A diagnostic ice-ocean model. Journal of Physics and Oceanography 17, 987-1015.
- Karklin, V. P. (1977)
- State and future of using of geliogeophysical factors for ice forecasts. Problemy Arctiki i Antarktiki 50, 45-48.
- Kowalik, Z. (1981)
- A study of the M2 tide in the ice-covered Arctic Ocean. Modeling, Identification and Control 2 (4), 201-223. INSPEC Compendex
- Kowalik, Z. and Murty, T. S. (1993)
- Numerical Modeling of Ocean Dynamics. World Scientific, Singapore, 481 p.
- Kowalik, Z. and Proshutinsky, A.Yu. (1994)
- The Arctic Ocean tides. In The Polar Oceans and Their Role in Shaping the Global Environment: The Nansen
Centenial Volume, Geophysics Monographic Series, vol. 85, eds O. Johannessen, R. D. Muench and J. E. Overland, pp. 137-158. AGU, Washington DC.
- Krutskikh, B. A. (1978)
- Main regularities of variation of the regime of Arctic Seas in natural hydrologic periods. Gidrometeoizdat, Leningrad, 91 p.
- McPhee, M. G. (1986)
- Analysis and prediction of short-term ice drift. In Proceedings of the Fifth International Offshore Mechanics and Arctic Engineering
Symposium, vol. 4, ASME, 385-39.
- Nansen, F. (ed.) (1902)
- The oceanography of the north polar basin. The Norwegian North Polar Expedition, 1893-1896, Scientific Results, Longmans, Green
and Co. vol. 3, 422 p.
- NCAR (1990)
- Compact disk of the National Meteorological Center Grid Point Data Set: Version II. General Information and user's guide. NCAR, 9 p.
- Piacsek, Allad S. R. and Warn-Varnas, A. (1991)
- Studies of the Arctic ice cover and upper ocean with a coupled ice-ocean model. Journal of Geophysical
Research 96, 4631-4650.
- Polyakov, I. V. (1994)
- Pers. comm. Arctic and Antarctic Research Institute, St. Petersburg, Russia.
- Ponomarev, V. I. (1977)
- Calculations of large-scale water circulation in the Arctic Basin using diagnostic model. Problemy Arctiki i Antarktiki 51, 65-75.
- Ponomarev, V. I. and Fel'zenbaum A. I. (1975)
- 2-D non-stationary model of wind driven circulation in the Arctic Basin. Okealologia XV (6), 17-23.
- Proshutinsky, A. Y. (1986)
- On the problem of calculating the storm surge fluctuations of sea level and water circulation in the Chukchi Sea. Meteorologiya i
Gidrologiya (Soviet Meteorology and Hydrology), vol. 1, translated from Russian by Allerton Press., Inc., pp. 54-61.
- Proshutinsky, A. Y. (1988)
- Modeling seasonal fluctuations of the level of the Arctic Ocean. Meteorologiya i Gidrologiya (Soviet Meteorology and Hydrology),
vol. 2, translated from Russian by Allerton Press., Inc., pp. 57-65.
- Proshutinsky, A. Y. (1993)
- The Arctic Ocean level oscillations. Hydrometeoizdat 216 p.
- Rothrock, D. A. (1975)
- The mechanical behavior of pack ice. Annual Review of Earth Planetary Sciences 3, 317-342. INSPEC
- Riedlinger, S. H. and Preller, R. H. (1991)
- The development of a coupled ice-ocean model for forecasting ice conditions in the Arctic. Journal of Geophysical
Research 96, 16, 955-16, 977.
- Semtner, A. J. (1976)
- Numerical simulation of the Arctic Ocean circulation. Journal of Physics and Oceanography 17, 1077-1099.
- Semtner, A. J., (1987)
- A numerical study of sea-ice and ocean circulation. Journal of Physics and Oceanography 6, 409-425.
- Shirshov, P. P. (1944)
- Scientific results of the drift of the Severnyi Polyus station. Report delivered at the General Assembly of the USSR Academy of Sciences on
14-17 Feb. 1944, Moskva, Izdatel'stvo Akademii Nauk SSSR, pp. 110-140.
- Shokal'skii, Yu. M. (1940)
- The G. Sedov, its drift, and the Geography of the Arctic Basin, Problemy Arktiki 2, 34-38.
- Shuleikin, V. V. (1941)
- Analysis of the drift of Severnyi Polyus station. DAN SSSR 31 (9), 886-892.
- Throndike, A. S., Colony, R. and Muñoz, E. A. (1982)
- Arctic Ocean Buoy program, Data report, 1 January 1982-31 December 1982, Polar Science Center,
University of Washington, 132 p.
- Treshnikov, A. F. (1959)
- Surface water in the Arctic Basin. Problemy Arktiki 7, 5-14.
- Treshnikov, A. F. and Baranov (1972)
- Water Circulation in the Arctic Basin. 145 p., Gidrometeoizdat, Leningrad, translated from Russian, Jerusalem, 1973.
- Treshnikov, A. F. and Baranov (1976)
- Structure of water circulation and dynamics of water budget in the northern polar region. Problemy Arktiki i Antarktiki 47,
93-100.
- Treshnikov, A. F., et al. (1976)
- Climatic trends in a dynamics of surface ice and water circulation in Amerasian subbasin (based on data obtained in 1949-1973.
Problemy Arktiki i Antarktiki 47, 74-91.
- Yanes, A. V. (1977)
- Year-to-year variability of water thermohaline conditions at the Faroe-Shetland canyon. Problemy Arktiki i Antarktiki 49, 34-38.
- Zubov, N. N. and Somov, M. M. (1940)
- Ice drift in the Northern Arctic Basin. Problemy Arktiki 2, 51-68.
Corresponding author (now at the Woods Hole Oceanographic Institution, USA). Tel.: +1-508-289-2796; fax: +1-508-457-2181; email: aproshutinsky@whoi.edu
adapted from: Marine Pollution Bulletin Volume 43, Issues 1-6, January-June 2001
Back to WHOI Arctic Group Projects
|
|