|
 |
Research > Science
Highlights > What Goes Down Must Come Up
What Goes Down Must Come Up
From space, the ocean may look like a big, calm bathtub. But
below its surface lies the ultimate dynamic environment—328
million cubic miles of water flowing, sinking, mixing, and rising.
It’s
as turbulent as another, more familiar fluid environment—the
atmosphere—but the action is hidden from view.
 |
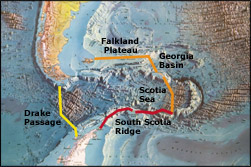 |
Data collected on five cruises between 1993 and 1999 (above: yellow, orange, and red tracks) showed evidence of intense turbulent mixing in the Southern Ocean. The mixing helps bring deep, dense waters back toward the surface. Oceanographers now hypothesize that much more mixing (below: red and yellow areas) may occur when the powerful Antarctic Circumpolar Current is disrupted as it flows over rough seafloor topography. (Illustration by Jayne Doucette)
|
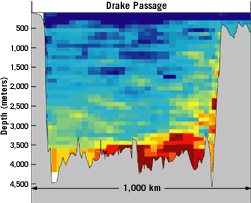 |
“We can observe the atmosphere because we live in it, rather
than just venturing on top of it,’’ said Bernadette Sloyan of
the WHOI Physical Oceanography Department.
“But we can’t look at ocean dynamics
directly to get information about what is happening over space
and time,” said her colleague, Kurt Polzin. Instead, physical
oceanographers work with measurements of thin slices of the
ocean at brief moments in time. They piece together snapshots,
envisioning the epic movie.
Small wonder, then, that one of the
most basic questions about ocean circulation remains a mystery.
The world’s oceans circulate like a conveyor belt of sinking
and rising waters: oceanographers know why cold waters sink
to the depths, but what makes them rise back to the surface
completing the loop?
It’s not an academic question, because
the oceans and atmosphere act as equal partners in a planetary
heating and ventilation system that transfers equatorial heat
to the poles. Together, they regulate Earth’s climate and keep
the planet habitable.
To understand and forecast changes in Earth’s
climate, scientists construct computer simulations of its complex
dynamics, said WHOI physical oceanographer Steven Jayne. But
the climate models have a fundamental blank spot: the “up” component
of the Ocean Conveyor.
Sinking ocean waters were relatively
easy to find and measure, because they are concentrated in a
few locations. Only in the North Atlantic and the Southern Ocean
around Antarctica do waters become both cold and salty (and
therefore dense) enough to plunge swiftly in a pipeline to the
deep.
But there’s no obvious pipeline coming back up. The cold water
spreads through the ocean depths, bounded by overlying layers
of lighter waters. It takes energy to break the boundaries between
layers, mix waters of different densities, and create a way
for denser waters to escape back upward.
“Mixing is dispersed
over areas thousands of times larger (than sinking) and happens
a thousand times more slowly,” Jayne said, which makes mixing
tough to locate, and harder still to measure.
 |
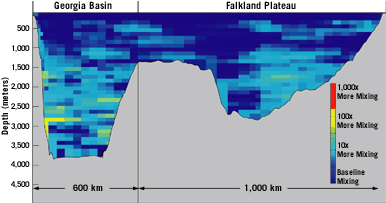 |
Data from Alberto C. Naveira Garabato, Kurt L. Polzin, Brian A. King, Karen J. Heywood, and Martin Visbeck in Science, Vol. 303, Jan. 9, 2004. |
Sloyan launched
a search to find where mixing might be occurring. She analyzed
salinity and temperature data from the World Ocean Circulation
Experiment, a multi-institution ambitious effort during the
1990s to collect global ocean measurements. She detected subtle
signs of mixing in the Southern Ocean—especially in regions
where the seafloor is marked by saw-toothed mountains, steep
slopes, and narrow chasms.
The Antarctic Circumpolar
Current whips around the continent at great speed and depth,
Sloyan said. It may bump into rough topography, like air currents hitting the Rockies, generating waves within the ocean’s interior. These internal waves can ripple away, overturning
density gradients and causing mixing. Currents surging through
a constrained passage, like a river through a narrow gorge,
may also force mixing.
Tides may also play a role, providing energy to move water back
and forth across seafloor topography. Jayne is exploring models
simulating how tides create internal waves that spur mixing
(see Doing the Wave).
Polzin narrows his investigations down to the microscale level,
often using a High-Resolution Profiler (HRP), an instrument
developed by WHOI scientists John Toole and Ray Schmitt and
equipped with sensors developed by former WHOI engineers Sandy
Williams, Neil Brown, and Tom Sanford. The HRP accurately measures
changes in water temperature, velocity, and conductivity (i.e.,
salinity) on scales ranging from tens of meters down to centimeters.
“We can reveal turbulence as small as this,” Polzin said, holding
his thumb and forefinger nearly together. “The HRP gives us
an ability to see and understand the ocean in new ways.”
Polzin is trying to find links between flows at disparate spatial
scales. “Small changes may not be recognized as important,”
he said “but they can initiate nonlinear, but coupled, changes
that are important.”
Today, many scientists see evidence that
ocean conditions may be approaching a tipping point that would
disrupt the delicately balanced ocean circulation systema
phenomenon that has abruptly rearranged global ocean currents
several times in Earth’s history and caused rapid, widespread
climate changes. Like a tiny cog in a colossal machine, centimeter-scale
mixing may prove a small but important piece that helps determine
whether the Ocean Conveyor shuts down or keeps running.
Lonny Lippsett (llippsett@whoi.edu)
|
|